Microinjection is one of the physical methods of gene transfer used to introduce DNA or other genetic materials directly into a cell using a small glass needle or micropipette.
This method allows efficient transfer and integration of desired genes into the host cell’s genome. It provides precise control over the delivery of desired materials making it a powerful tool in various research areas.
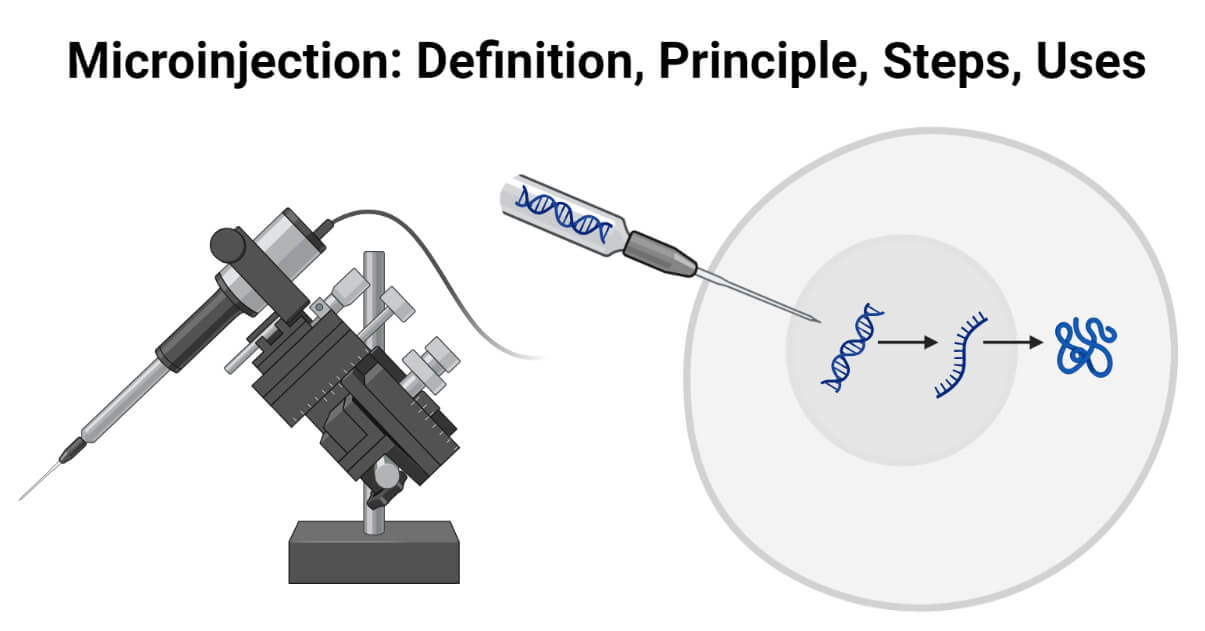
Dr. Marshall A. Barber introduced the concept of DNA microinjection during the early 19th century. Since then, it has continually evolved to keep up with the advancements in biomedical fields. It has applications in diverse areas such as transgenics, animal cloning, human infertility treatment, genetic engineering, and genome editing.
Interesting Science Videos
Principle of Microinjection
The principle of microinjection is based on the direct delivery of genetic material into individual cells using a fine glass needle called a micropipette, a positioning device known as a micromanipulator, and a microinjector. The process is performed under a powerful microscope. The genetic material is delivered into the cell by applying hydrostatic pressure to release a fluid containing the DNA through the micropipette. The small tip diameter of the micropipette and the precise movements enabled by the micromanipulator allows the precise delivery of desired materials.
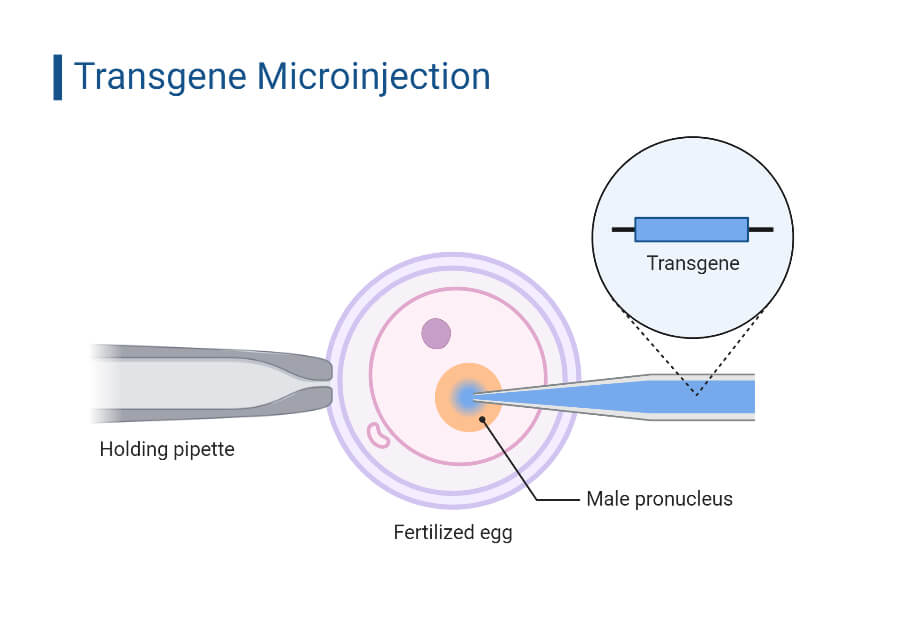
Steps in Microinjection
- First, the glass micropipette or needle is prepared by heating and stretching the glass to form a fine tip at the heated end. The resulting tip size is usually around 0.5 mm in diameter, resembling an injection needle.
- The entire process of delivering foreign materials using microinjection is performed under a powerful microscope for precise manipulation and observation.
- The cells to be microinjected are placed in a container.
- A holding pipette is placed near the target cell. It uses gentle suction to hold the cell during the injection process.
- The micropipette, containing the desired contents, is mounted on a micromanipulator, which allows precise positioning and movement of the pipette. The micropipette is lowered near the cell.
- The micropipette is carefully inserted into the cell membrane, either into the cytoplasm or the nucleus, depending on the target location for the genetic material. The contents of the micropipette are then injected into the cell by applying hydrostatic pressure using a microinjector. The pressure forces the fluid containing the genetic material to be released through the micropipette into the cell.
- After delivering the contents into the cell, the empty micropipette needle is slowly and carefully withdrawn from the cell, minimizing damage to the cell and its membrane. Proper technique and high-quality micropipettes help minimize cell death during this step.
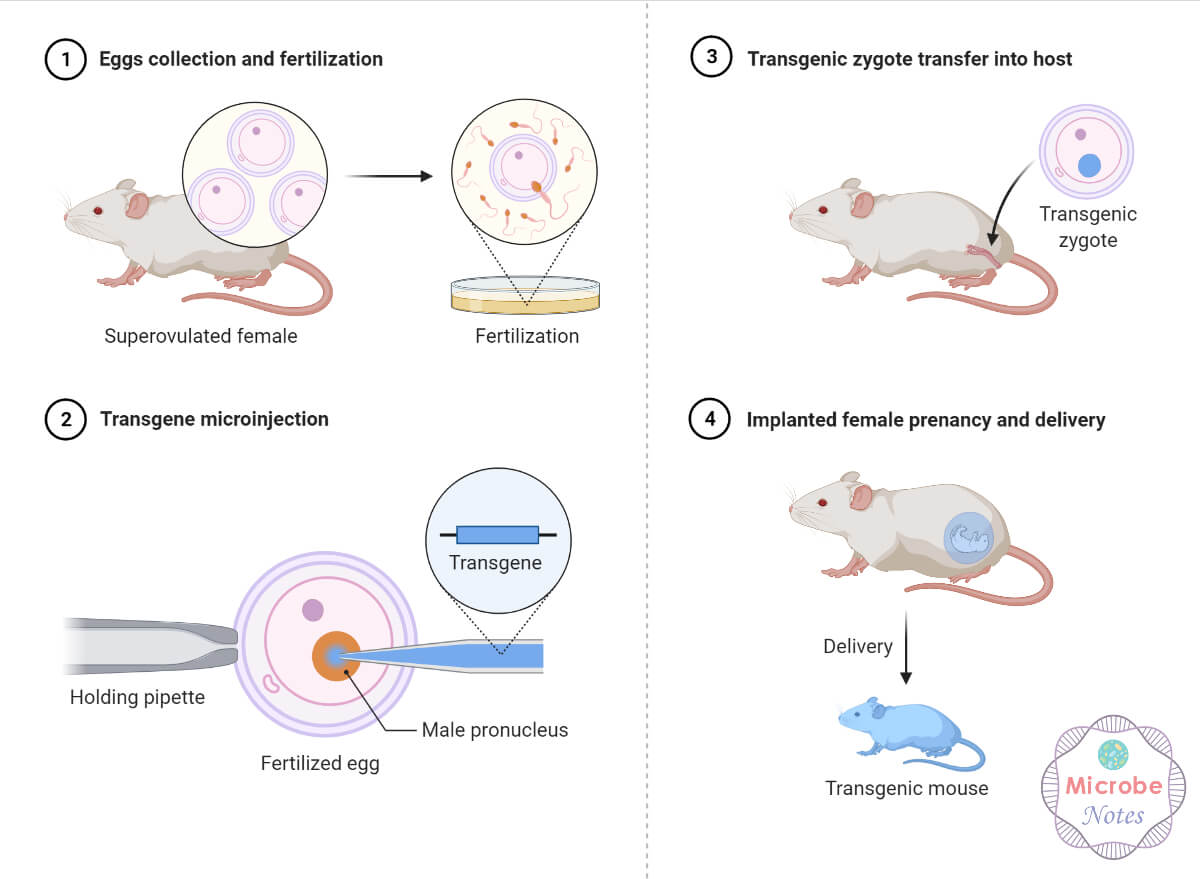
Applications of Microinjection
Some major applications of microinjection are:
- Microinjection is commonly used in the production of transgenic animals, introducing foreign DNA into fertilized eggs to study gene function and create disease models.
- Microinjection was used to produce the first chimeric transgenic mice. Chimeric animals have been useful in studying embryonic development, tissue transplantation, and understanding cell lineage.
- In the field of in vitro fertilization, microinjection is used in intracytoplasmic sperm injection (ICSI), allowing successful fertilization in cases of male infertility, and leading to term pregnancies and healthy births. In ICSI, individual sperm with motility defects are microinjected directly into isolated oocytes.
- Microinjection is also used in somatic cell nuclear transfer (SCNT) to create genetically identical copies of an organism by transferring a somatic cell into an enucleated oocyte.
- Microinjection has been widely used in various studies, including human embryo research and injection of mitotic cells.
- In neuroscience, microinjection is useful for working with primary cultured human neurons, delivering proteins, peptides, and cDNA constructs into the cytosol of human neurons which is difficult to perform using other gene transfer techniques.
Advantages of Microinjection
- Unlike other gene transfer methods, microinjection does not require the use of selection markers such as antibiotic-resistance genes. This makes the process easier and removes the need for additional steps to identify and isolate transformed cells.
- Microinjection allows precise delivery of materials in terms of volume and timing, which can be challenging with other methods like electroporation or transfection.
- Injected cells can be easily identified by co-injecting a marker dye or fluorescently labeled proteins.
- Microinjection requires less protein preparation compared to electroporation, making it advantageous for experiments involving less abundant or expensive proteins and peptides.
- Microinjection is less stressful to cells, reducing cell death that is commonly observed with chemical transfection or viral infection methods.
Disadvantages of Microinjection
- Microinjection requires vehicle controls to assess potential effects on cell viability and to ensure accurate results.
- The technical expertise required to master the microinjection method and maintain cell viability is another limitation of this method.
- The use of alternative techniques like transfection, infection, and electroporation has become more popular compared to microinjection for delivering non-permeable foreign materials into single cells.
- Manual microinjection is labor-intensive and time-consuming, making it less practical for large-scale experiments or high-throughput applications.
- Microinjection typically involves injecting a small population of cells within a larger cell culture, which can limit scalability.
- Microinjection is not an appropriate method for transferring genetic material into a large number of cells for techniques like Western blotting or purification.
- Direct microinjection of certain proteins, like membrane proteins or neurotransmitter receptors, is challenging.
References
- Carter, M., & Shieh, J. (2015). Gene Delivery Strategies. Guide to Research Techniques in Neuroscience, 239–252. doi:10.1016/b978-0-12-800511-8.00011-3
- Dean, D. A. (2013). Microinjection. Brenner’s Encyclopedia of Genetics, 409–410. doi:10.1016/b978-0-12-374984-0.00945-1
- Feramisco, J., Perona, R., & Lacal, J. C. (1999). Needle Microinjection: A Brief History. Microinjection, 9–15. doi:10.1007/978-3-0348-8705-2_1
- https://player.uacdn.net/lesson-raw/H4MR25C5TIRWG6HWIYOL/pdf/6351074197.pdf
- https://www.ncbi.nlm.nih.gov/books/NBK19648/
- Jinturkar, K. A., Rathi, M. N., & Misra, A. (2011). Gene Delivery Using Physical Methods. Challenges in Delivery of Therapeutic Genomics and Proteomics, 83–126. doi:10.1016/b978-0-12-384964-9.00003-7
- Korzh, V., & Strähle, U. (2002). Marshall Barber and the century of microinjection: from cloning of bacteria to cloning of everything. Differentiation, 70(6), 221–226. doi:10.1046/j.1432-0436.2002.700601.x
- Xu, W. (2018). Microinjection and Micromanipulation: A Historical Perspective. Microinjection, 1–16. doi:10.1007/978-1-4939-8831-0_1