Interesting Science Videos
What is spectroscopy?
- Spectroscopy is the study of the interaction between light and matter where the absorption and emission of light or other radiation by the matter are studied and measured.
- Spectroscopy mainly deals with the dispersion of light and other radiations that is caused by an object which allows the study of various properties of the object.
- The measurement in spectroscopy is a function of the wavelength of the radiation being observed.
- Spectroscopy has been widely exploited as it allows the determination of composition, physical and electronic structure to be determined of various particles of molecular or atomic levels.
What is a spectrometer?
- The spectrometer is a scientific instrument that is used to measure the variation or differences in various properties caused by an object over a particular range.
- The property observed by a spectrometer varies with the type of spectrometer being used.
- NMR spectrometer measures the variation in nuclear resonance frequencies, mass spectrometer measure the difference in mass to charge ratio whereas an optical spectrometer measures the variation in the electromagnetic radiation.
- Based on the measurement of these variations, different properties of particles can be measured and observed.
What is a spectrophotometer?
- The spectrophotometer is a particular type of spectrometer that measures the interaction (absorption, reflection, scattering) of electromagnetic radiation from a sample or the emission (fluorescence, phosphorescence, electroluminescence) of electromagnetic radiation by various sample.
- It is also termed electromagnetic spectrometer as it deals with the measurement of different properties of light and its interaction with matter.
- These are commonly used in laboratories to measure the concentration of various samples on the basis of total light absorbed by the sample.
What is a spectroscope?
- A spectroscope or optical spectrometer is a device that measures different properties of light over a specific range in the spectrum used for the analysis of various objects.
- The property measured is mostly the intensity of light, although polarization of light is also measured under some conditions.
- Spectroscopes are commonly used in studies regarding astronomy and chemistry for the analysis of various samples.
- Traditionally, prisms were used as spectroscopes, however, nowadays, diffraction gratings, mobile slit, and photodetectors are used.
- These are mostly used to deduce the chemical composition of objects based on the radiation produced by different objects.
What is a spectrograph?
- The spectrograph is a scientific instrument that detects different light and separates them by their wavelength or frequencies which are recorded by multi detectors.
- These are mostly used for obtaining and recording the astronomical spectrum.
- Spectrographs are used for astronomical studies as telescopes.
- In a spectrograph, the light rays transfer into the spectrograph through the telescope which is provided with a mirror that functions to makes all light rays parallel to each other.
- The rays then reach the diffraction grating that disperses the light into different wavelengths which are passed to the detectors for the analysis of the individual wavelengths.
- These are highly useful to analyze the incoming light from various astronomical objects for the analysis of the chemical composition of those objects.
What are spectra?
- Spectra, singular spectrum, in optics, are the colors observed when white light is dispersed through a prism.
- Spectrum refers to the range of various variables associated with light and other waves.
- In light, the electromagnetic spectrum is the most commonly used. The electromagnetic spectrum includes the range of frequencies of electromagnetic radiation that are used to characterize the distribution of electromagnetic radiation absorbed or emitted by an object.
- Besides, the mass spectrum is also used in spectroscopy based on the ion abundance as a function of the mass to charge ratio.
- Electron spectrum is another spectrum used in physics that is the number or intensity of particle beam depending on the particle energy.
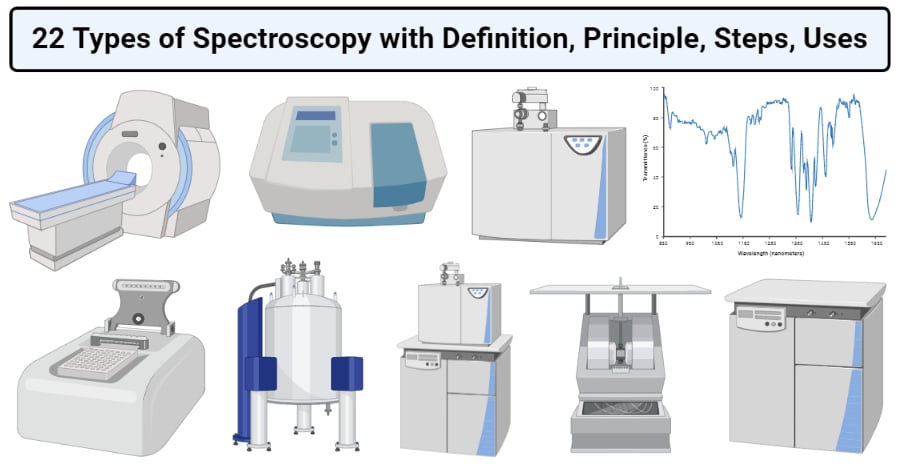
1. Absorption spectroscopy
Absorption spectroscopy is a spectroscopic technique that measures the frequency of wavelength of absorbed light as a result of the interaction between the light and a sample.
Principle of Absorption spectroscopy
- Absorption spectroscopy is based on the principle that materials have an absorption spectrum which is a range of radiation absorbed by the material at different frequencies.
- The absorption spectrum of materials depends on the atomic and molecular composition of that material.
- The frequency of light radiation absorbed by a material is dependent on the energy difference between the two energy states of the molecules.
- The absorption results in the formation of an absorption line, which, together with other lines, form an absorption spectrum.
- Thus, when a photon with sufficient energy reaches an object, the energy is absorbed by the electrons causing them to bump into a higher energy state.
- The amount of photon (radiation) absorbed results in an absorption spectrum which can then be measured in terms of absorbance.
- The absorbance of a sample is dependent on the number of excited electrons which in turn is dependent on the concentration of molecules in the sample.
Steps of Absorption spectroscopy
- Solvent liquid and the sample solution are taken in two transport vessels, also termed as cuvettes.
- The vessel with solvent liquid is then placed in the spectrometer to determine the light loss due to scattering and absorbance by the solvent. Any absorbance observed in this process is to be subtracted from the absorbance of the sample.
- The cuvette with the sample solution is then placed in the spectrometer.
- The absorbance of the sample is noted in different frequencies which usually ranges from 200-800 nm.
- A similar spectrum is formed from a different concentration of the samples.
- A graph of the absorbance measured against the concentration of the sample is plotted, which can then be used for the determination of the unknown concentration of the sample.
Uses of Absorption spectroscopy
- Absorption spectroscopy is used to determine the presence of a particular substance in a sample and then to quantify the present substance.
- This technique has been applied in remote sensing, which allows the determination of the concentration of hazardous substances without the direct interaction of the instrument and the sample.
- Absorption spectroscopy is also used for the determination of the atomic and molecular structure of various substances.
2. Astronomical spectroscopy
Astronomical spectroscopy is the study of astronomical structures by using the principle of spectroscopy for the measurement of the electromagnetic spectrum, which is radiated from stars or other celestial bodies.
Principle of Astronomical spectroscopy
- The spectrum produced by the light coming from celestial objects is not as smooth as the spectrum of white light.
- Both the absorption and emission spectrum of light is produced by passing the light through a diffraction grating.
- The light, when dispersed, forms absorption and emission lines which are dependent on the energy levels of electrons in atoms and molecules of the object present in the source.
- Based on the absorption of radiation at a particular wavelength, a graph is plotted between the wavelength and the flux of the absorption and emission lines.
- Most of these lines are formed due to the presence of metals. Thus, depending on the height and depth of these lines, the abundance of these metals can be quantified.
Steps of Astronomical spectroscopy
- In astronomical spectroscopy, the incoming light from various stars is passed through a telescope into the spectroscope.
- As the light reaches the diffraction grating of the spectroscope, the light is dispersed into different wavelengths.
- The dispersed wavelengths land on the photodetectors which analyze the nature of the wavelengths.
- The detectors form the flux scale of the spectrum depending on the wavelength by comparison with observations of standard stars.
Uses of Astronomical spectroscopy
- The absorption of light from the stars reveals various properties of stars like chemical composition, temperature, density, mass, and relative motion.
- The study of the stellar spectrum helps in the study of galaxies and the composition of galaxies.
- Astronomical spectroscopy can also be used for the determination of the motion of stars and galaxies through the Doppler effect and redshift.
3. Atomic absorption spectroscopy
Atomic absorption is an analytical technique utilizing the principle of spectroscopy for the quantitative determination of chemical elements.
Principle of Atomic absorption spectroscopy
- Atomic absorption spectroscopy utilizes the principle that free electrons generated in an atomizer absorb radiation of different wavelengths.
- The free electrons absorb UV or visible light, causing the electrons to transfer to higher energy orbits.
- During this process, the absorption spectrum is released, which is detected by the photodetectors.
- The absorption spectrum formed allows the quantification of free electrons in the gaseous state of the matter.
- The amount of photon (radiation) absorbed results in an absorption spectrum which can then be measured in terms of absorbance.
- The absorbance of a sample is dependent on the concentration of molecules in the sample.
Steps of Atomic absorption spectroscopy
- The liquid sample is mixed with a particular volume of spirit which is added to a flask which is then vaporized into a gas by a fuel-rich acetylene-nitrous oxide flame.
- A lamp is set with the necessary wavelength as a light source.
- The gas formed from the liquid sample is then passed through a detector that detects the absorbance of the atoms in the gas.
- A similar process is performed for the detection of absorbance of solvent bank and standard solution.
- A graph is plotted for the absorbance against the concentration of the molecules in the sample.
Uses of Atomic absorption spectroscopy
- Atomic absorption spectroscopy can be used for the quantitative and qualitative determination of metallic elements in biological systems.
- This also helps in the detection of metals as an impurity in alloys and other mixtures.
- Atomic absorption spectroscopy has been utilized for the purification of environmental samples like water and soil.
- Detection of metals in pharmaceutical products and oil products can also be done by this method.
4. Circular dichroism spectroscopy
Circular dichroism spectroscopy is a type of light absorbance spectroscopy that measures the differences in the absorbance of right and left polarized light.
Principle of Circular dichroism spectroscopy
- Left- and right-handed polarized components of the incident light are absorbed differently by the sample, which yields a difference in the absorption coefficients. This difference is termed circular dichroism.
- Circularly-polarized light rays will travel through an optically active medium with different velocities due to the different indices of refraction for right- and left-circularly polarized light.
- Optically active chiral molecules will preferentially absorb one direction of the circularly polarized light.
- The peptide bonds in protein act as chromophores. The peptide bonds are the optically active chiral molecules of protein, and the number of chromophores is proportional to the magnitude of absorption.
- Thus, the magnitude of absorption is then used for the verification of the adopted secondary structure of proteins.
Steps of Circular dichroism spectroscopy
- The sample is placed in a transport vessel with buffers which is then placed in the spectrometer.
- In the spectrometer, left and right circularly polarized light passes through the sample in an alternating fashion.
- The photomultiplier detector in the spectrometer produces a voltage proportional to the circular dichroism (the difference between the absorbance of left and right polarized light) of the resultant beam emerging from the sample.
- The circular dichroism of the sample is then compared with standard proteins to determine the differences in the secondary structure of the proteins.
Uses of Circular dichroism spectroscopy
- The primary application for CD spectroscopy is the verification of the assumed secondary structure of the protein. This method allows the detection of percentages of α-helix and β-sheet in proteins base on their circular dichroism.
- Circular dichroism spectroscopy can also be used to monitor changes of secondary structure within a sample over time.
- This technique can also be used to compare two macromolecules to detect the differences in the structure of the molecules.
- It can also be used for the analysis of pharmaceutical products to ensure that they are still present in the folded active conformations.
5. Electrochemical impedance spectroscopy (EIS)
Electrochemical impedance spectroscopy is an advanced electrochemical technique that measures the impedance of a system by applying different AC potential frequencies.
Principle of Electrochemical impedance spectroscopy (EIS)
- Electrochemical Impedance Spectroscopy is a technique that measures how a new material or device impedes the flow of electricity.
- This is done by applying an AC signal through the electrodes connected to the sample.
- AC voltage at different frequencies is applied to a sample, and the electrical current is measured.
- A Nyquist plot is generated from the response of frequency to the electrical impedance by plotting the impedance on the y-axis and the frequency on the x-axis.
- The instrument applies an alternating field voltage to the sample and measures the current response.
- The real and imaginary components of impedance are calculated by determining the phase shift and change in amplitude at different frequencies.
Steps of Electrochemical impedance spectroscopy (EIS)
- A test module is first hooked to the EIS that confirms that the wires connected to the system are hooked correctly, and all the parts are working correctly.
- To start the flow of current in the system, the Zplot software is operated on the computer. The required parameters are set, and the AC amplitude is set to 10mV. The initial frequency is set to 1× 106 and the final frequency to 100Hz.
- The Zview software is then operated to view the results.
- The electrodes are then removed from the test module.
- The sample is prepared and assembled into the test furnace located in the hood fume.
- The electrodes are attached to the assembly, and the EIS is operated as the same previous procedures.
Uses of Electrochemical impedance spectroscopy (EIS)
- The impedance of a sample can be used to determine the population of microorganisms when bacteria grow in a sample.
- EIC can also be used to screen for cancer tissues where the impedance of the electric current changes as the cell structure and size changes.
- This has also been used to study the application of layers of chemicals, polymers, or coatings to electrodes which provide useful enhancements in terms of electron transfer and sensitivity.
6. Electron spin resonance (ESR) spectroscopy
Electron Spin Resonance (ESR) also known as Electron Magnetic Resonance (EMR) or Electron Paramagnetic Resonance (EPR) is a type of absorption spectroscopy in which radiations having a frequency in the microwave region (0.04 – 25 cm) are absorbed by paramagnetic substances resulting in transitions between magnetic energy levels of electrons having unpaired spins.
Principle of ESR spectroscopy
- Electron spin resonance spectroscopy is based on the fact that the atoms, molecules, or ions with unpaired electrons exhibit magnetic properties because of the electron spin.
- When a molecule or compound is placed in a magnetic field, the spin of the unpaired electrons can align in two different states.
- If the spin is aligned in the direction of the magnetic field, it results in a lower energy state. In contrast, the opposite alignment of the magnetic field results in a higher energy state.
- As a result, the unpaired electron can move between these two energy states by either absorbing or emitting photons of energy resulting in a resonance condition.
- The spectrum produced during this absorption is passed to the detectors for the analysis of the electronic structures of the sample.
Steps of ESR spectroscopy
- The ESR instrument is turned on and warmed up for 30 minutes. The necessary parameters are set in the ESR.
- Calibration is performed by adding an empty ESR tube to ensure no background signals from the tube or the instrument is present.
- The sample is then placed in the ESR tube, and the ESR spectrum is measured.
Uses of ESR spectroscopy
- Electron spin resonance spectroscopy is one of the essential methods for the study of transition metal-containing metalloproteins.
- This method also allows the study of the denaturation of proteins and protein folding.
- ESR also helps determine the rate of catalysis of different metals.
- It has also been widely applied in the field of biological research of quantitative and qualitative analyses of reactive nitrogen species (RNS) and reactive oxygen species (ROS).
7. Emission spectroscopy
Emission spectrometry is a spectrometric technique that measures the wavelengths of photons emitted by atoms or molecules while transitioning from a high energy level to a lower energy state.
Principle of Emission spectroscopy
- When electrons or compounds are heated either on a flame or by an electric heater, they emit energy in the form of light.
- The light emitted from the compound is passed into a spectrometer then disperses the light into separate wavelengths.
- Each element forms a different atomic spectrum that indicates that an atom can radiate only a certain amount of energy.
- Each element emits a set of discrete wavelengths that is characteristic to it based on its electronic structure, and from these wavelengths, the elemental composition of the sample can be determined.
Steps of Emission spectroscopy
- The solution containing the sample is heated either in a flame or with an electric heater.
- The solvent evaporates first, whereas the finely divided solid particle remains in the center of the flame along with other molecules and ions.
- This causes the excitation of electrons which produces the radiation of a specific wavelength.
- The radiation is passed through the spectrometer where the monochromator disperses the light into different wavelengths.
- Detectors detect the wavelengths in the spectroscope.
- A graph of wavelength against the concentration is plotted to determine the concentration of the sample.
Uses of Emission spectroscopy
- Emission spectroscopy has wide applications in agricultural and environmental analysis along with industrial analysis for the detection of metals and alloys.
- It can also be used in the determination of lead in petrol.
- This has been applied for the determination of an equilibrium constant of ion exchange resins.
8. Energy dispersive spectroscopy
Energy dispersive spectroscopy, also known as Electron dispersive X-ray spectroscopy, is an analytical technique for the elemental or chemical characterization of the sample by using the X-ray interaction with the sample.
Principle of Energy dispersive spectroscopy
- The principle of energy dispersive spectroscopy is similar to emission spectroscopy except that the excitation of electrons is brought about by X-ray beams.
- The compound in a sample has electrons in the ground state bound to the nucleus.
- When X-rays are focused on a compound, the electrons in the ground level get excited and reach an inner shell.
- This results in an electron-hole in the outer shell which is then filled by another electron from an outer high energy shell.
- The difference in the energy between the high-energy shell and the low-energy shell is released in the form of X-ray beams.
- The energy dispersive spectroscopy determines the energy and number of X-ray beams released from the sample.
- The difference in energy between two shells is characteristics of the atomic structure of the sample, which helps in the determination of the composition of the sample.
Steps of Energy dispersive spectroscopy
- Two samples of known and unknown concentrations are taken in a transport vessel, also termed as a cuvette.
- The vessels are then placed, one after the other, in the spectrophotometer that is provided with an excitation source which can either be an electron beam or an x-ray beam and detectors.
- The spectrophotometer is operated that passes an x-ray through the sample.
- The photosensitive detectors present in the spectrophotometer detect the x-ray passing through the sample, which is then converted into voltage signals.
- The voltage signals then enter the pulse processor that measures the signals and passes them to an analyzer for data display and further analysis.
Uses of Energy dispersive spectroscopy
- Energy-dispersive X-ray spectroscopy (EDS) as a part of electron microscopy has been widely used in many research areas as it provides precise information on the chemical composition of subcellular structures.
- EDS has been used in medical diagnostics as X-ray microanalysis may be useful in defining criteria for malignant tissue formation.
- The application of electron dispersive spectroscopy in environmental analysis is a useful tool to estimate the risk of polluted air in terms of human health.
- Droplets of pesticides (0.5 µl – 1 µl) at varying concentrations deposited on plant surfaces can also be analyzed by this method.
9. Fluorescence spectroscopy
Fluorescence spectroscopy is a type of electromagnetic spectroscopy that utilizes the fluorescence produced by objects in a sample which is not necessarily in the visible range of the spectrum.
Principle of Fluorescence spectroscopy
- The principle of fluorescence spectroscopy is similar to emission spectroscopy, where the transition of electrons from one state to another causes the emission spectrum.
- Fluorescence is an emission phenomenon where a transition from a higher to a lower energy state is accompanied by radiation.
- Only molecules in their excited forms can emit fluorescence; thus, they have to be brought into a higher energy state prior to the emission phenomenon.
- The emitted radiation appears as a band spectrum because there are many closely related wavelength values dependent on the vibrational and rotational energy levels attained.
- The fluorescence spectrum of a molecule is independent of the wavelength of the exciting radiation and has a mirror image relationship with the absorption spectrum.
- The probability of the transition from the electronic excited to the ground state is proportional to the intensity of the emitted light.
- The fluorescence properties of a molecule are determined by features of the molecule itself and thus help in the determination of the composition of the molecules.
Steps of Fluorescence spectroscopy
- Two samples of known and unknown concentrations are taken in a transport vessel, also termed as a cuvette.
- The vessels are then placed, one after the other, in the spectrofluorimeter that is provided with light source and detectors.
- The spectrofluorimeter is operated that passes light of a particular wavelength through the sample.
- The photosensitive detectors present in the spectrophotometer detect the light passing through the sample, which is then converted into digital values.
- A graph of the fluorescence measured against the concentration of the sample is plotted, which can then be used for the determination of the unknown concentration of the sample.
Uses of Fluorescence spectroscopy
- Fluorescence spectroscopy is used in biomedical, medical, and chemical research for the analysis of organic compounds.
- This has also been used to differentiating malignant tumors from benign tumors.
- Atomic fluorescence spectroscopy can also be used for the detection of metals in various environmental samples like air, water, and soil.
- In analytical chemistry, fluorescence detectors are used along with HPLC.
10. Fourier-transform infrared (FTIR) spectroscopy
Fourier-transform infrared spectroscopy is a technique used for the detection of the infrared spectrum of absorption and emission of molecules in solid, liquid, or gaseous phase. The term Fourier-transform has been used because this process requires a mathematical process termed Fourier transform to convert the raw data into the actual spectrum.
Principle of FTIR spectroscopy
- Fourier-transform infrared spectroscopy uses the same principle of dispersive spectroscopy except that instead of focusing a beam with a single frequency, this technique uses a beam with multiple frequencies.
- This technique then measures how much of that beam is absorbed by the sample.
- Similarly, a second beam with different frequencies is used for sample processing, and the data is collected.
- This process is repeated a number of times over a short period of time.
- All the data obtained is then given to a computer that works backward to determine the absorption at each wavelength.
- The spectrometer has a special structure termed Michelson interferometer which consists of a certain number of mirrors, one of which moves towards the light source.
- With the movement of the mirror, each wavelength of light is periodically blocked and transmitted due to wave interference.
- As a result of this, the beam coming out of the interferometer has a different spectrum each time.
Steps of FTIR spectroscopy
- The sample is placed in the FTIR spectrometer. The sample should be thin enough for the IR rays to pass through.
- The device directs the IR towards the sample and measures how much and which frequency of the beam is absorbed by the sample.
- With the help of the reference database, the sample is identified.
Uses of FTIR spectroscopy
- FTIR spectroscopy is commonly used for the analysis of organic, polymeric, and even inorganic compounds.
- This technique allows the determination of the chemical composition of various samples.
- FTIR has been used with gas chromatography for the detection of substances separated from the chromatographic technique.
- FTIR can directly detect the polarity of the given site while investigating hydrophobic membrane environments.
11. Gamma-ray spectroscopy
Gamma-ray spectroscopy is an analytical technique used for the study of the energy spectrum of gamma rays formed from radioactive objects in a sample.
Principle of Gamma-ray spectroscopy
- During radioactive decay, gamma decay is also seen in radioactive substances.
- In gamma decay, the nucleons in the nucleus found in discrete levels move towards the lower energy state, and the energy difference is released in the form of a gamma-ray.
- The gamma rays are then passed through detectors that convert the radiation into electric signals for the computer.
- The detection and quantification of gamma rays help to determine the nature of the source.
- By comparing the measured energy to the known energy of gamma-rays produced by radioisotopes, the identity of the emitter can be determined.
Steps of Gamma-ray spectroscopy
- The counting chamber is opened using the handle. The interior of the counting chamber is usually shielded with lead and then lined with copper to reduce background radiation caused by x-rays emitted by the lead.
- The sample container is placed carefully on top of the detector, and the counting chamber is closed.
- Background counting is performed to determine if contamination or natural radiation is present in amounts that would interfere with sample data.
- Further analysis is done through computing systems.
Uses of Gamma-ray spectroscopy
- Gamma-ray spectroscopy is exclusively used for the determination of nuclear structure, nuclear transitions, and nuclear reactions.
- This has also been employed for astronomical research like fort detection of water on other planets.
- Gamma-ray spectroscopy has been utilized for the elemental and isotopic analysis of airless bodies in the solar system, especially the moon and mars.
12. Infrared (IR) spectroscopy/ Vibrational spectroscopy
Infrared spectroscopy, also termed vibrational spectroscopy, is a technique that utilizes the interaction between infrared and the sample.
Principle of IR spectroscopy/ Vibrational spectroscopy
- The wavelength utilized for the analysis of organic compounds ranges from 2,500 to 16,000 nm, with a corresponding frequency range from 1.9×1013 to 1.2×1014 Hz.
- These rays don’t have enough energy to excite the electrons, but they do, however, cause the vibrational excitation of covalently bonded atoms or groups.
- The vibration observed in the atoms is characteristic of these atoms and thus helps in the detection of the molecules.
- The infrared spectrum is the fundamental measurement obtained in infrared spectroscopy.
- The spectrum is a plot of measured infrared intensity versus wavelength (or frequency) of light.
- IR Spectroscopy measures the vibrations of atoms, and based on this; it is possible to determine the functional groups.
Steps of IR spectroscopy/ Vibrational spectroscopy
- The IR spectrometer is turned on and allowed to warm up for 30 minutes.
- The unknown sample is taken, and its appearance is recorded.
- The background spectrum is collected to remove the spectrum obtained from natural reasons.
- A small amount of sample is placed under the probe by using a metal spatula.
- The probe is set in place by twisting it.
- The IR spectrum of the unknown sample is obtained. The process is repeated, if necessary, to get a good quality spectrum.
- The absorption frequencies that indicate the functional groups present are recorded.
- The obtained spectrum is analyzed to determine the probable identification of the unknown sample.
Uses of IR spectroscopy/ Vibrational spectroscopy
- Infrared spectroscopy has been widely used for the characterization of proteins and the analysis of various solid, liquid, and gaseous samples.
- IR spectroscopy can be used for the detection of functional groups which helps in the identification of molecules and their composition.
- Applications of IR spectroscopic techniques allow identifying molecular changes due to bodily changes, understanding of the molecular mechanism of various diseases, and identifying specific spectral biomarkers that can be used in diagnosis.
13. Magnetic resonance spectroscopy
Magnetic resonance spectroscopy is a diagnostic technique used alongside magnetic resonance imaging (MRI) which measures biochemical changes that take place in different parts of the body.
Principle of Magnetic resonance spectroscopy
- Magnetic resonance spectroscopy analyzes the hydrogen atoms present in the sample.
- The nucleus of the atom is excited by applying a magnetic field that causes the spinning of the nucleus in a particular direction.
- The difference in the energy of the different directions of spin results in a spectrum characteristic to the nucleus of the atom.
- The frequencies of the spectrum are determined, which helps to analyze the concentration of various metabolites.
- By measuring the concentration of the metabolites, and comparing it to the normal concentration in various cells, determination of the type of tissue found in the body can be determined.
Steps of Magnetic resonance spectroscopy
- The MR instrument is turned on and warmed up for 30 minutes. The necessary parameters are set in the magnetic resonance spectrometer.
- Calibration is performed by adding an empty MR tube to ensure no background signals from the tube or the instrument is present.
- The sample is then placed in the MR tube, and the magnetic resonance spectrum is measured.
Uses of Magnetic resonance spectroscopy
- Magnetic resonance spectroscopy enables doctors and chemists to obtain biochemical information regarding tissue in the body which helps in the diagnostic process.
- MR spectroscopy is used to assist the MRI technique generally performed for diagnostic purposes in hospitals.
- It is increasingly used in research for medical projects.
- This technique has also been used in creating profile serum samples metabolically from patients diagnosed with hepatocellular carcinoma (HCC).
14. Mass spectroscopy
Mass spectroscopy is a type of spectroscopic technique that helps to identify the amount and type of chemicals present in the sample by analyzing the mass to charge ratio of the ions.
Principle of Mass spectroscopy
- Mass spectroscopy is based on the principle that when a sample is bombarded with electrons, the molecules in the compounds are ionized into ions.
- The separation of ions is dependent on their mass to charge ratio. For most ions, the charge is one which means that the ratio is simply the molecular mass of the ion.
- The ions are then subjected to electric and magnetic fields which causes deflection of the ions. Ions with a similar charge to mass ratio show similar deflection.
- The relative abundance of each of such ions is then detected with the help of the detectors.
- The mass spectrum is formed by plotting the relative abundance of the ions against the ratio of mass to charge.
- The spectrum can then be used for the determination of the elemental configuration of the sample, the masses of the particle or molecules, and the chemical structure of the sample.
Steps of Mass spectroscopy
- 200 µl of the sample is mixed with 1.8 ml of 65% nitric acid.
- The mixture is then added to the water bath at 50°C overnight.
- The tubes are then cooled down to room temperature, and the sample is diluted by adding 8 ml distilled water to obtain nitric acid concentration below 20%.
- The sample is then added to the spectroscope and run.
- The results are obtained through the software on the computer in the form of the mass spectrum.
Uses of Mass spectroscopy
- Mass spectroscopy is a valuable tool to quantify known materials.
- It also allows the identification of unknown compounds and determination of the structure and chemical composition of various substances.
15. Molecular spectroscopy
Molecular spectroscopy is a type of spectroscopy that utilizes the interaction between molecules and electromagnetic radiation to determine the structural composition of samples.
Principle of Molecular spectroscopy
- Molecular spectroscopy involves the interaction of materials with electromagnetic radiation in order to produce an absorption pattern (i.e. a spectrum) from which structural or compositional information can be deduced.
- The molecular spectrum is formed when the molecules move from one energy state to another as a result of interaction with different electromagnetic radiation.
- The mechanisms involved are similar to atoms, but the process is more complicated.
- The interaction between different nuclei and electrons takes place in molecules that are not observed in atoms.
- These interactions can be electronic, rotational, or vibrational. Based on the type of interactions, different spectrometric techniques can be applied for the analysis of the compounds.
- Similarly, either the absorption or emission spectrum can be utilized based on the type of interactions being exploited.
Steps of Molecular spectroscopy
- Different types of spectrometer systems like emission spectrometer and Fourier-transform spectrometer can be used for molecular spectroscopy.
- All these systems include a source of radiation, a sample, and a system for detecting and analyzing the sample.
- The sample is added to the spectrometer where the radiation source focuses on it.
- Two samples of known and unknown concentrations are taken in a transport vessel, also termed as a cuvette.
- The vessels are then placed, one after the other, in the spectrophotometer that is provided with light source and detectors.
- The spectrophotometer is operated that passes light of a particular wavelength through the sample.
- The photosensitive detectors present in the spectrophotometer detect the light passing through the sample, which is then converted into digital values.
- A graph of the absorbance measured against the concentration of the sample is plotted, which can then be used for the determination of the unknown concentration of the sample.
Uses of Molecular spectroscopy
- Molecular spectroscopy allows the analysis of the atomic and molecular structures of various compounds.
- It also helps in the determination of gas composition as well as the composition of other compounds.
16. Mossbauer spectroscopy
Mossbauer spectroscopy is a technique based on the Mossbauer effect discovered by Rudolf Mössbauer which utilizes the spectrum formed by the absorption or emission of nuclear gamma rays in solid particles.
Principle of Mossbauer spectroscopy
- Mossbauer effect states that the recoil energy associated with absorption or emission of a photon can be described by the conservation of momentum.
- In solids, the nuclei of the atoms are not free but are bound to a particular lattice.
- When a nucleus in a solid particle emits or absorbs a gamma-ray, the energy always lost occurs in discrete packets called phonons which are the quantized vibration of the crystal lattice.
- In some cases, however, the no phonons might be released, and the conservation of momentum in such cases is maintained by the momentum of the lattice as a whole.
- Thus, gamma rays emitted by one nucleus can be completely absorbed by a sample containing nuclei of the same isotope, and this absorption can be measured.
- The measured Mossbauer spectrum can be used to detect the presence of radioactive samples.
Steps of Mossbauer spectroscopy
- A sample is placed inside the spectrometer.
- A beam of gamma radiation is then passed through the sample. The atoms in the radiation source emitting the gamma rays must have the same isotope as the atoms in the sample absorbing them.
- The detector then measures the intensity of the beam transmitted through the sample.
- The spectrum formed is then analyzed for various purposes.
Uses of Mossbauer spectroscopy
- Mossbauer spectroscopy is a sensitive technique that can detect even subtle changes in the nuclear environment.
- It provides precise information about the chemical, structural and magnetic properties of the various objects.
- It has also been used in geology to detect the composition of meteors and moon rocks.
- This technique has been highly used in bioorganic chemistry for the study of iron-containing proteins and enzymes.
17. Nuclear magnetic resonance (NMR) spectroscopy
Nuclear magnetic resonance spectroscopy is a technique that utilizes the magnetic resonance of the nucleus to determine the structure of various compounds.
Principle of NMR spectroscopy
- Nuclear magnetic resonance spectroscopy is based on the fact that the nuclei in the atoms, molecules, or ions are charged and have spin.
- When a molecule or compound is placed in a magnetic field, the spin of the nucleus can align in two different states.
- If the spin is aligned in the direction of the magnetic field, it results in a lower energy state, whereas the opposite alignment of the magnetic field results in a higher energy state.
- As a result, the nucleus can move between these two energy states by either absorbing or emitting photons of energy resulting in a resonance condition.
- The spectrum produced during this absorption is passed to the detectors for the analysis of the nuclear structures of the sample.
Steps of NMR spectroscopy
- The NMR instrument is turned on and warmed up for 30 minutes. The necessary parameters are set in the NMR.
- Calibration is performed by adding an empty NMR tube to ensure no background signals from the tube or the instrument is present.
- The sample is then placed in the NMR tube, and the NMR spectrum is measured.
Uses of NMR spectroscopy
- NMR spectroscopy is used in quality control.
- It is also used in the determination of the content and purity of the sample while detecting the structure of the compounds.
- Solid-state NMR spectroscopy is performed to determine the molecular structure of solids.
- It can also be used for the determination of protein structure.
18. Photoelectron spectroscopy
Photoelectron spectroscopy, also known as photoemission spectroscopy, is a spectrometric technique that measures the electrons released from a substance as a result of the photoelectric effect to determine the binding energies of the electrons.
Principle of Photoelectron spectroscopy
- Photoelectron spectroscopy utilizes the principle of the photoelectric effect.
- The sample is exposed to UV rays that induce photoionization of the substances.
- The emitted photoelectrons have energies that are characteristic of their original energy states and the vibrational and rotational level of the electrons.
- The energy of these electrons is used to determine the binding energy of the electrons in the atoms by the given formula:
BE= hν- KE where hν is the incident radiation, and KE is the energy of the emitted photoelectrons.
- A graph of BE against KE is then plotted.
- The peaks in the spectrum indicate the electrons in different subshells of an atom. The lowest peaks indicate the valence electrons, whereas the highest peaks correspond to the core electrons.
Steps of Photoelectron spectroscopy
- The sample is placed in the spectrometer, which is exposed to the ionizing radiation from the radiation source.
- The emitted electrons strike the detectors, which then converts the energy into an electric signal.
- The signal is transferred to the analyzer to obtain the analog data from the signal.
- Then a graph is plotted of the kinetic energy of the emitted electrons and the binding energy.
- Based on the peaks formed in the graph, the unknown element can be detected.
Uses of Photoelectron spectroscopy
- Electron spectroscopy is sensitive to every element except hydrogen and helium and thus can play an important role in all phases of chemistry.
- Since the electronic energy levels of atoms are sensitive to their environment, this technique is the best available for determining the oxidation states of atoms in molecules.
- From the photoelectron spectra, one can obtain the relative ratios of different elements and different atoms of the same element.
- This technique is sensitive to many essential areas of research such as corrosion studies, catalysis, semiconductor studies, adsorbed gas work, and electrode studies.
19. Raman spectroscopy
Raman spectroscopy is an analytical technique that provides detailed information about the chemical structure, polymorphism, crystallinity, and molecular interactions within a compound.
Principle of Raman spectroscopy
- A Raman spectrum arises from the analysis of scattered light from the sample.
- The largest part of an incident light beam passes through the sample (transmission). A small part is scattered isotropically, i.e. uniformly in all directions, and possesses the same wavelength as the incident beam.
- The Raman spectrum arises from the fact that a tiny proportion of light scattered by the sample will have a different frequency than the incident light.
- As a result of the incident photon, the molecules move to an exciting rotational-vibrational-electronic state, and the photons are emitted from the sample.
- In order to maintain the energy of the system after the molecule moves to a higher state, the scattered photons shift to a new state.
- The energy difference between the new state and the original state of the scattered photon is equal to the difference in energy between the two vibrational states of the molecules.
- The criterion for a peak to appear in the Raman spectrum is a change in polarizability of the molecule during the transition.
- The depolarization is calculated as the ratio of two intensities with perpendicular and parallel polarization with respect to the incident beam.
Steps of Raman spectroscopy
- Mostly aqueous solutions are used the required laser is turned on after selecting the appropriate wavelength being used.
- The spectrometer is then calibrated by using a reference sample by using appropriate exposure energy and time.
- The sample is then placed under the microscope while focusing on the layer to be investigated.
- A range of wavenumbers is scanned by the monochromator, which results in the Raman spectrum.
- The data is then investigated by appropriate software, and the analysis is made based on the peaks formed on the spectrum.
Uses of Raman spectroscopy
- Raman spectroscopy is employed over infrared spectroscopy as it allows the analysis of the sample in aqueous conditions.
- This technique can also be used to determine the chemical composition and structure of various samples.
20. UV spectroscopy
UV spectroscopy is a type of absorption spectroscopy where UV lights are absorbed by the electrons that causes them to excite to a high energy state.
Principle of UV spectroscopy
- In UV spectroscopy, the UV rays passed to the sample are absorbed by the electrons, which increases the energy of the system.
- This causes the excitation of an electron from a lower energy state to a higher energy state.
- This excitation forms an absorption spectrum that can be detected by the detectors in the spectrometer.
- The amount of photon (radiation) absorbed results in an absorption spectrum which can then be measured in terms of absorbance.
- The absorbance of a sample is dependent on the number of excited electrons which in turn is dependent on the concentration of molecules in the sample.
Steps of UV spectroscopy
- Two samples of known and unknown concentrations are taken in a transport vessel, also termed as a cuvette.
- The vessels are then placed, one after the other, in the spectrophotometer that is provided with light source and detectors.
- The spectrophotometer is operated that passes light of a particular wavelength through the sample.
- The photosensitive detectors present in the spectrophotometer detect the light passing through the sample, which is then converted into digital values.
- A graph of the absorbance measured against the concentration of the sample is plotted, which can then be used for the determination of the unknown concentration of the sample.
Uses of UV spectroscopy
- UV spectroscopy is a technique used for the detection of impurities in organic substances.
- This can also be used for the quantitative determination of compounds that can absorb UV radiation.
- It can also be used for the study of the kinetics of a reaction where the UV rays are passed through the reaction cell, and the changes in absorbance are studied.
21. Ultraviolet and visible (UV/Vis) spectroscopy
Ultraviolet and visible spectroscopy is an absorption spectroscopy technique which uses the radiation in the UV range and the adjacent visible range of the electromagnetic radiation.
Principle of UV/Vis spectroscopy
- UV/Vis spectroscopy is based on the principle that materials produce an absorption spectrum which is a range of absorbance resulting from the radiation absorbed by the material at different frequencies.
- The absorption spectrum of materials depends on the atomic and molecular composition of that material.
- The frequency of light radiation absorbed by a material is dependent on the energy difference between the two energy states of the molecules.
- The absorption results in the formation of the absorption line, which, together with other lines, form an absorption spectrum.
- The incident light in this spectrometer is in the range of UV and visible spectrum of the electromagnetic spectrum.
- Thus, when a photon with sufficient energy reaches an object, the energy is absorbed by the electrons causing them to bump into a higher energy state.
- The amount of photon (radiation) absorbed results in an absorption spectrum which can then be measured in terms of absorbance.
- The absorbance of a sample is dependent on the number of excited electrons which in turn is dependent on the concentration of molecules in the sample.
Steps of UV/Vis spectroscopy
- Solvent liquid and the sample solution are taken in two transport vessels, also termed as cuvettes.
- The vessel with solvent liquid is then placed in the spectrometer to determine the light loss due to scattering and absorbance by the solvent. Any absorbance observed in this process is to be subtracted from the absorbance of the sample.
- The cuvette with the sample solution is then placed in the spectrometer.
- The absorbance of the sample is noted in different frequencies which usually ranges from 200-800 nm.
- A similar spectrum is formed from the different concentrations of the samples.
- A graph of the absorbance measured against the concentration of the sample is plotted, which can then be used for the determination of the unknown concentration of the sample.
Uses of UV/Vis spectroscopy
- Qualitative analysis may be performed in the UV/Vis regions to identify certain classes (proteins and nucleic acids) of compounds both in the pure state and in biological mixtures.
- This type of spectroscopy is used for the quantification of biological samples either directly or via colorimetric assays.
22. X-ray photoelectron spectroscopy
X-ray photoelectron spectroscopy is a sensitive, quantitative spectroscopic technique based on the photoelectric effect used for the identification of electrons inside a compound, on the surface as well as the chemical state and the electronic configuration of the compound.
Principle of X-ray photoelectron spectroscopy
- Photoelectron spectroscopy utilizes the principle of the photoelectric effect.
- The sample is exposed to X-rays with specific wavelengths that induce photoionization of the substances.
- The emitted photoelectrons have energies that are characteristic of their original energy states and the vibrational and rotational level of the electrons.
- The energy of these electrons is used to determine the binding energy of the electrons in the atoms by the given formula:
BE= hν- KE where hν is the incident radiation, and KE is the energy of the emitted photoelectrons.
- A graph of BE against KE is then plotted.
- The peaks in the spectrum indicate the electrons in different subshells of an atom. The lowest peaks indicate the valence electrons, whereas the highest peaks correspond to the core electrons.
Steps of X-ray photoelectron spectroscopy
- The sample is placed in the spectrometer, which is exposed to the ionizing radiation from the radiation source.
- The emitted electrons strike the detectors, which then converts the energy into an electric signal.
- The signal is transferred to the analyzer to obtain the analog data from the signal.
- Then a graph is plotted of the kinetic energy of the emitted electrons and the binding energy.
- Based on the peaks formed in the graph, the unknown element can be detected.
Uses of X-ray photoelectron spectroscopy
- X-ray photoelectron spectroscopy is commonly used for the measurement of composition, chemical state, and electronic configuration of various organic and inorganic substances.
- This also assists in the surface analysis of various compounds.
References
- Wilson, K., Walker, J. (2018). Principles and Techniques of Biochemistry and Molecular Biology. Eighth edition. Cambridge University Press: New York.
- Randviir, Edward & Banks, Craig. (2013). Electrochemical impedance spectroscopy: An overview of bioanalytical applications. Analytical methods. 5. 1098-1115. 10.1039/c3ay26476a.
- Kohno M. (2010). Applications of electron spin resonance spectrometry for reactive oxygen species and reactive nitrogen species research. Journal of clinical biochemistry and nutrition, 47(1), 1–11. https://doi.org/10.3164/jcbn.10-13R
- Wyroba E, Suski S, Miller K, Bartosiewicz R. Biomedical and agricultural applications of energy-dispersive X-ray spectroscopy in electron microscopy. Cell Mol Biol Lett. 2015;20(3):488-509. DOI:10.1515/cmble-2015-0028
- Ayca Dogan Mollaoglu, Ipek Ozyurt and Feride Severcan (November 5th 2018). Applications of Infrared Spectroscopy and Microscopy in Diagnosis of Obesity, Infrared Spectroscopy – Principles, Advances, and Applications, Marwa El-Azazy, IntechOpen, DOI: 10.5772/intechopen.81216. Available from: https://www.intechopen.com/books/infrared-spectroscopy-principles-advances-and-applications/applications-of-infrared-spectroscopy-and-microscopy-in-diagnosis-of-obesity
- Tognarelli, J. M., Dawood, M., Shariff, M. I., Grover, V. P., Crossey, M. M., Cox, I. J., Taylor-Robinson, S. D., & McPhail, M. J. (2015). Magnetic Resonance Spectroscopy: Principles and Techniques: Lessons for Clinicians. Journal of clinical and experimental hepatology, 5(4), 320–328. https://doi.org/10.1016/j.jceh.2015.10.006
- Goodman B.A. (1994) Molecular spectroscopy: introduction and general principles. In: Wilson M.J. (eds) Clay Mineralogy: Spectroscopic and Chemical Determinative Methods. Springer, Dordrecht
- L. Cheng, J.W. Prather II & Thomas A. Carlson(1975) Applications of Photoelectron Spectroscopy to Analytical Chemistry, C R C Critical Reviews in Analytical Chemistry, 5:1, 37-85, DOI: 10.1080/10408347508542680.
Wonderful information provided