The mitochondria of eukaryotic cells perform the intricate process of cellular respiration. It includes converting food into adenosine triphosphate (ATP), the cell’s primary energy source.
Oxidative phosphorylation, a procedure that takes place within the inner mitochondrial membrane, is one of the crucial processes in cellular respiration.
The process, elements, and control of oxidative phosphorylation will be examined in this article, offering insight into its critical function in energy production.
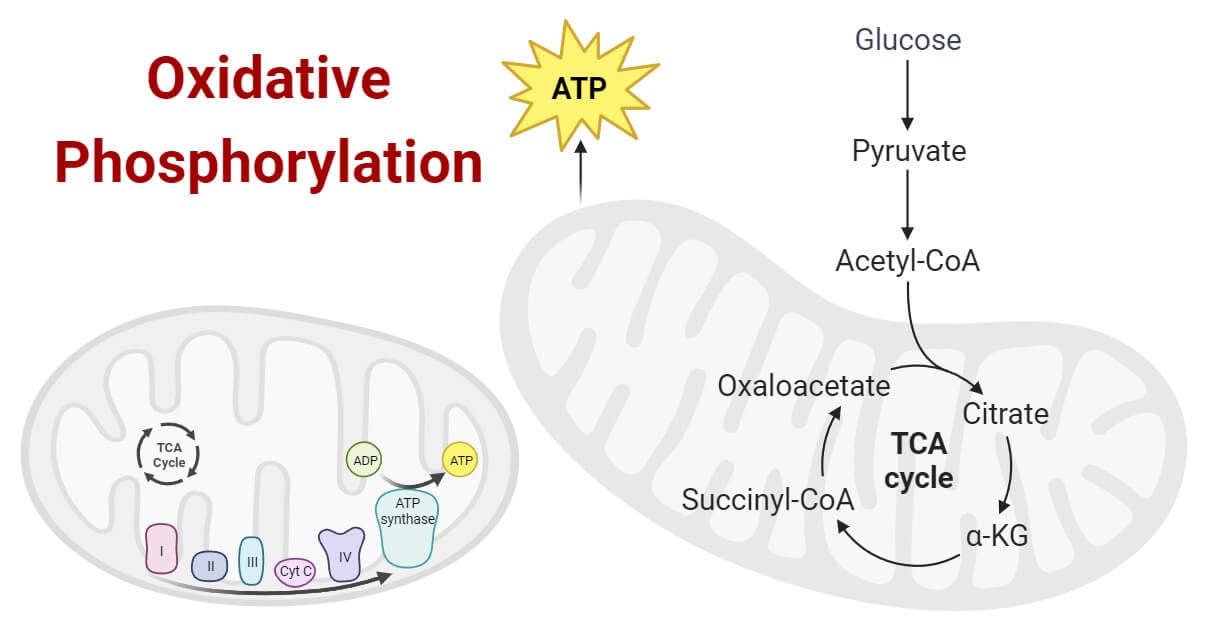
Interesting Science Videos
Overview of Cellular Respiration
Cellular respiration is crucial to comprehending the context of cellular respiration in order to comprehend oxidative phosphorylation.
- In this metabolic route, glucose or other organic molecules are broken down to produce carbon dioxide, water, and ATP.
- Glycolysis, the citric acid cycle (sometimes referred to as the Krebs cycle or TCA cycle), and oxidative phosphorylation are the three main steps of cellular respiration.
- The bulk of ATP is produced at the final phase, oxidative phosphorylation.
Anatomy of the Mitochondrion
The mitochondrion is a double-membraned organelle with a unique structure that facilitates oxidative phosphorylation.
- Its inner membrane contains invaginations known as cristae, which provide a large surface area for the assembly of protein complexes involved in electron transport and ATP synthesis.
- The mitochondrial matrix, enclosed by the inner membrane, contains enzymes necessary for the citric acid cycle.
What is an Electron Transport Chain?
The electron transport chain (ETC) is a series of protein complexes embedded in the inner mitochondrial membrane.
- These complexes, labeled I to IV, play a crucial role in oxidative phosphorylation. Electrons from NADH and FADH2, generated during glycolysis and the citric acid cycle, are shuttled through the ETC.
- This movement of electrons releases energy, which is harnessed to pump protons (H+) across the inner mitochondrial membrane, establishing an electrochemical gradient.
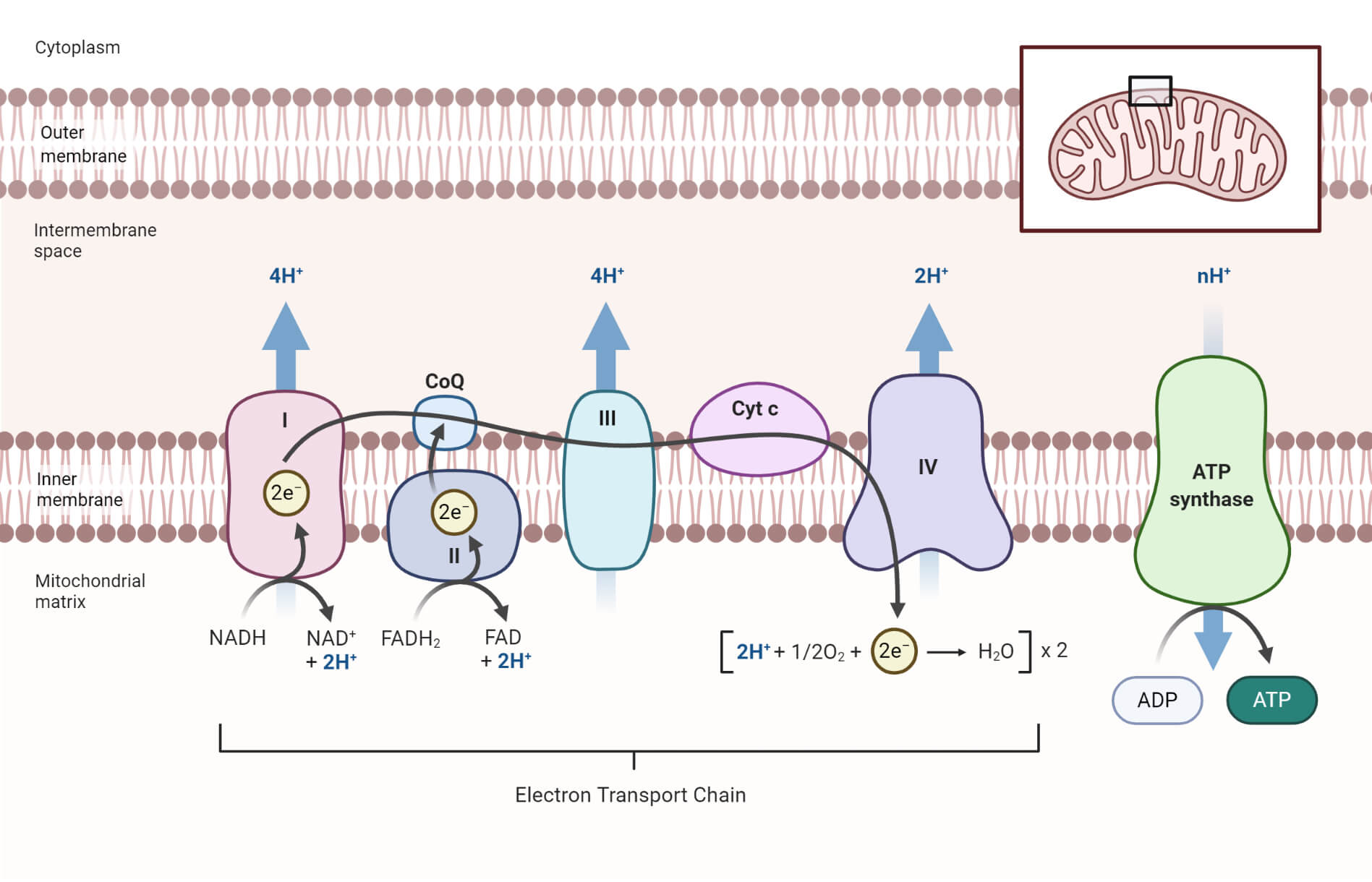
Chemiosmosis and ATP Synthesis
The electrochemical gradient generated by the ETC drives chemiosmosis, the process by which ATP is synthesized. ATP synthase, located in the inner mitochondrial membrane, acts as a molecular turbine, utilizing the flow of protons down their concentration gradient to generate ATP. This coupling of proton flow with ATP synthesis is known as oxidative phosphorylation. The ATP produced is then utilized by the cell for various energy-requiring processes.
Proton Pumping and Electron Carriers
- The process of proton pumping is essential to oxidative phosphorylation.
- Proton pumps actively transfer protons from the matrix to the intermembrane space as electrons move through the ETC.
- These pumps transport protons by using the energy created by the transfer of electrons.
- Additionally, the effective transmission of electrons across protein complexes in the ETC is made possible by electron transporters like ubiquinone and cytochrome C.
Regulation of Oxidative Phosphorylation
- The regulation of oxidative phosphorylation is a complex process that ensures ATP production meets cellular energy demands.
- Several factors influence its activity, including the availability of oxygen, the concentrations of ADP and ATP, and the proton gradient across the inner mitochondrial membrane.
- Regulatory mechanisms, such as feedback inhibition and the control of electron carrier availability, modulate oxidative phosphorylation to maintain energy homeostasis.
Factors Affecting Oxidative Phosphorylation
Inhibitors
Electron transport and phosphorylation are closely correlated in physiological settings. Anomalies in oxidative phosphorylation might result from substances that interfere with phosphorylation processes or that alter electron transport. We will now discuss four variables that impact oxidative phosphorylation.
- A chemical known as a respiratory chain inhibitor prevents electron transport at a specific point in the respiratory chain and prevents the oxidation process.
- Some respiratory chain inhibitors, including rotenone, phenoxymycin A, and barbital, prevent the transfer of electrons from NADH to CoQ by binding to iron-sulfur proteins in NADH-Q reductase.
- Antimycin A and dimercaptopropanol are two chemicals that prevent the transport of electrons between Cytb and Cytc1.
- Cytochrome oxidase is inhibited by cyanide, azide, H2S, and C0, which prevents electrons from moving from carbon to oxygen.
Oxidative Phosphorylation Inhibitors
These chemicals obstruct electron transport and directly interfere with ATP synthesis. It is impossible for hydrogen ions to return from the proton channel when oligomycin and dicyclohexylcarbonyldiimide are combined with the F0 unit of ATP synthase. This results in an incomplete phosphorylation process, which precludes the oxidative phosphorylation of intact mitochondria.
Uncoupling Agent
- The uncoupling agent separates the two coupling processes of electron transfer and ATP synthesis.
- Such compounds only inhibit the formation of ATP but do not affect the electron transfer process. So the free energy generated by electron transfer is converted into heat energy, which excessively uses oxygen and fuel substrates.
- Such agents cause the electron transfer to lose normal control, resulting in excessive utilization of oxygen and fuel substrates, and energy is not stored.
- A typical uncoupler is 2,4-dinitrophenol (DNP). Because DNP is a fat-soluble substance, it can move freely in the mitochondrial membrane.
- When it enters the matrix, it can release H+. Return to the cytosol side. The H+ can be combined to eliminate the transmembrane gradient of H+ so that the Uncoupling agent: This substance divides the two coupling processes of ATP production and electron transport.
- Such substances do not interfere with the electron transfer mechanism; they merely prevent the production of ATP.
- As a result, electron transfer’s free energy is transformed into heat energy, which consumes an excessive amount of oxygen and fuel substrates.
- By causing the electron transport to deviate from normal regulation, such agents result in an increased usage of oxygen and fuel substrates and no energy storage.
- The uncoupler 2,4-dinitrophenol (DNP) is a common one. DNP is able to move about easily in the mitochondrial membrane since it is a fat-soluble chemical.
- It is able to release H+ when it enters the matrix. Back on the cytosolic side now. The transmembrane gradient of H+ can be removed by combining the H+.
The rate of oxidative phosphorylation is mostly governed by ADP in normal organisms. The rate of oxidative phosphorylation increases after being transported into the mitochondria when the body utilizes more ATP; otherwise, when there is insufficient ADP, the rate of oxidative phosphorylation slows down. This control enables the rate of ATP synthesis to change in response to physiological demands.
Thyroid hormone
- Thyroid hormone has the ability to activate Na+-K+ATPase on the cell membrane of several tissues, speed up the breakdown of ATP into ADP and Pi, and increase the amount of ADP in mitochondria.
- This decreases the ATP/ADP ratio and speeds up the rate of oxidative phosphorylation.
- The body produces more heat and oxygen as the rate of ATP synthesis and breakdown increases, which increases the basal metabolic rate, one of the most significant clinical indicators for hyperthyroidism patients.
Mitochondrial DNA Mutation
- Because mitochondrial DNA (mtDNA) lacks a protein protection and damage repair mechanism and has a bare circular double helix shape, it is prone to mutations caused by oxidative phosphorylation.
- Thirteen oxidative phosphorylation-related proteins are encoded by mtDNA.
- Therefore, mtDNA mutations may have an impact on the oxidative phosphorylation pathway, which might lead to a reduction in ATP production and a variety of illnesses.
Environmental Factors
Environmental factors can impact oxidative phosphorylation and cellular respiration. Exposure to toxins, pollutants, or certain drugs can disrupt the electron transport chain and impair ATP synthesis. Additionally, alterations in nutrient availability, such as fasting or a high-fat diet, can influence oxidative phosphorylation efficiency. Understanding these interactions can provide insights into the effects of environmental factors on cellular metabolism and overall health.
Diseases and Disorders
Impairments in oxidative phosphorylation can lead to various diseases and disorders. Mitochondrial diseases, resulting from genetic mutations affecting the components of oxidative phosphorylation, can manifest as neurological, muscular, or metabolic disorders. Reactive oxygen species (ROS), byproducts of oxidative phosphorylation, can cause oxidative stress and contribute to aging and various pathological conditions, including cancer and neurodegenerative diseases.
Clinical Implications and Therapeutic Approaches
- Understanding the intricacies of oxidative phosphorylation has important clinical implications.
- Diagnostic tests, such as measuring oxygen consumption or mitochondrial DNA analysis, can aid in the diagnosis of mitochondrial disorders.
- Therapeutic approaches for mitochondrial diseases often involve managing symptoms and providing supportive care, as there is currently no cure.
- However, ongoing research focuses on potential treatments targeting mitochondrial dysfunction, such as the development of antioxidant therapies and gene therapies.
Conclusion
An essential step in cellular respiration, oxidative phosphorylation is crucial for producing ATP, the cell’s energy currency. This procedure effectively generates ATP by chemiosmosis by capturing the energy from proton pumping and electron transfer. Understanding cellular metabolism, identifying mitochondrial diseases, and creating viable therapies all depend on an understanding of the processes, elements, and control of oxidative phosphorylation. New understandings of cellular energetics, environmental impacts, and treatment options for numerous illnesses linked to mitochondrial malfunction should be revealed as this field of study continues to develop.
References
- Biochemistry, Oxidative Phosphorylation – https://www.ncbi.nlm.nih.gov/books/NBK553192/
- Oxidative phosphorylation – https://www.khanacademy.org/science/ap-biology/cellular-energetics/cellular-respiration-ap/a/oxidative-phosphorylation-etc
- Oxidative Phosphorylation – https://www.cusabio.com/pathway/Oxidative-Phosphorylation.html