Interesting Science Videos
What is cellulose?
Cellulose is an organic polysaccharide composed of a linear chain of hundreds of β-linked D-glucose units.
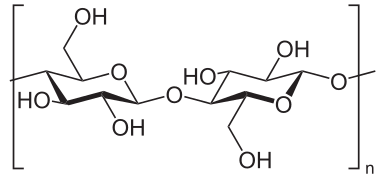
Figure: Cellulose, a linear polymer of D-glucose units (two are shown) linked by β(1→4)-glycosidic bonds. Image Source: NEUROtiker.
- Cellulose is the most abundant extracellular structural polysaccharide or organic polymer of all biomolecules in the biosphere.
- Cellulose is present in all land plants but is completely lacking in meat, egg, fish, and milk. It is, however, not metabolized by the human system.
- It is the most widely distributed carbohydrate of the plant kingdom that comprises about 50% of all the carbon in vegetation.
- Cellulose occurs in the cell walls of plants where it contributes in a major way to the structure of the organism.
- All cellulose-synthesizing organisms, including bacteria, algae, tunicates, and higher plants, have cellulose synthase proteins, which catalyze the polymerization of glucan chains.
- Even if the human body cannot digest cellulose, it acts as a source of fiber.
- In nature, cellulose is a source of food to a wide variety of organisms including bacteria, fungi, plants, and protists as well as a wide range of invertebrate animals, like insects, crustaceans, annelids, molluscs, and nematodes.
- The mechanical strength of the plant cell is attributed to the structural properties of cellulose as it can retain a semi-crystalline state of aggregation even in the aqueous environment.
- Cellulose is a homopolymer of a glucose derivative, and thus, it acts as a great source of fermentable sugar.
- Cellulose is cultivated in the form of energy crops for the production of ethanol, ethers, acetic acid, etc.
- The abundance of cellulose is due to the constant photosynthetic cycles in higher plants, synthesizing about 1000 tons of cellulose.
- Cellulose is a fibrous, rigid, white solid, insoluble in water but soluble in ammoniacal cupric hydroxide solution.
- Although insoluble in water, cellulose absorbs water and adds to the bulk of the fecal matter, and facilitates its removal.
Structure of cellulose
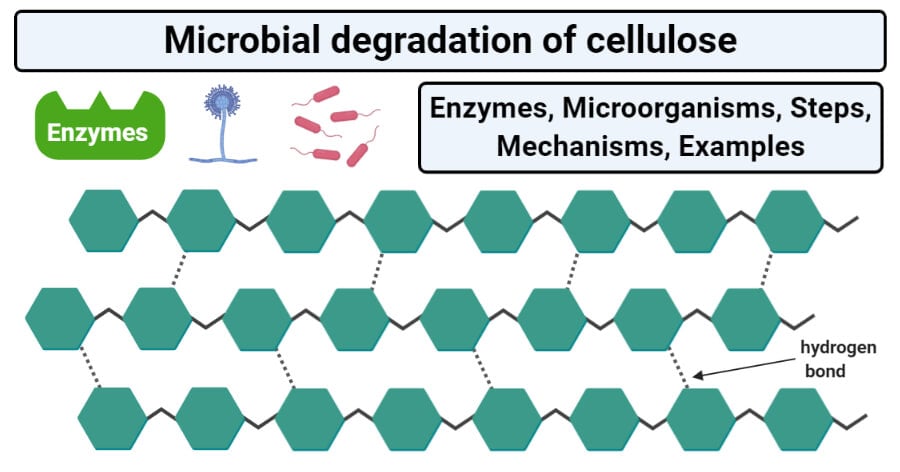
- The molecular weight of cellulose ranges between 200,000 and 2,000,000, thus corresponding to 1,250–12,500 glucose residues per molecule.
- Cellulose consists of a D-glucose unit at one end with a C4-OH group as the non-reducing end, and the terminating group is C1-OH as the reducing end.
- The bond is formed by taking out a molecule of water from the glycosidic OH group on carbon atom 1 of one β-D-glucose molecule and the alcoholic OH group on carbon atom 4 of the adjacent β-D-glucose molecule.
- Anhydrocello biose is the repeating unit of cellulose.
- The overall structure of cellulose is a result of the binding of adjacent cellulose chains and sheets by hydrogen bonds and van der Waals forces, resulting in a parallel alignment.
- This results in the crystalline structure of cellulose with straight, stable supramolecular fibers of great tensile strength and low accessibility.
- The structure of cellulose resembles in structure with amylose except that the glucose units are linked together by β-1, 4-glucoside linkages.
- The β-1, 4-glycosidic linkage in the structure creates a linear glucan chain where every other glucose residue is rotated at 180° to the neighbor.
- The cellulose molecule is very stable, with a half-life of 5–8 million years for b -glucosidic bond cleavage at 25°C.
- Cellulose is of different types on the basis of their structure and accessibility; crystalline and non-crystalline, accessible and non-accessible.
- Most of the cellulose found in wood is highly crystalline with about 65% crystalline regions. The rest of the structure has a lower packing density, resulting in an amorphous or non-crystalline structure.
- Accessibility of cellulose is used to define the availability of cellulose to water and microorganisms. The surface of crystalline cellulose is mostly accessible, whereas the rest of the structure is non-accessible.
What are cellulases?
Cellulases refer to a group of enzymes that catalyze the breakdown of cellulose to form oligosaccharides, cellobiose, and glucose.
- These enzymes represent a class of enzymes that are produced by fungi and bacteria that assist in the hydrolysis of cellulose.
- Cellulose is an important group of enzymes that play an essential role both in industries as well as in nature.
- In nature, cellulases are involved in the global carbon cycle by degrading insoluble cellulose into soluble forms.
- Cellulases are structurally distinct and diverse, which hydrolyzes a single substrate, cellulose, even though there are seven different protein folds within the family.
- The complete enzymatic system of cellulase consists of three enzymes, exo- β -1, 4-glucanases, endo- β -1, 4-glucanases, and β -1, 4-glucosidases.
- These enzymes act sequentially in a synergistic system to bring about the breakdown of cellulose to generate a utilizable energy source in the form of glucose.
- Cellulases are generally divided into four major classes on the basis of their mode of action; exoglucanases, endoglucanases, β-glucosidases, and cellobiohydrolases.
- These enzymes are different in their structure and the mode of action, however, in some cases, the enzymes can act sequentially to produce the desired end product.
- Cellulases are also different in different organisms like the fungal, and bacterial cellulases significantly differ in their structure and functions.
- Fungal cellulases, unlike bacterial cellulases, consist of a carbohydrate-binding module (CBM) at the C-terminal joined by a short polylinker region to the catalytic domain at the N-terminal.
Microorganisms involved in cellulose degradation (cellulolytic microorganisms)
A broad spectrum of cellulolytic microorganisms, mainly fungi, and bacteria, have been identified over the years. The structure and mode of action of the cellulases produced by different microorganisms are also different.
Cellulolytic Fungi
- Fungi are among the most active agents of decomposition of organic matter in general and of the cellulosic substrate in particular.
- Cellulase-producing fungi are widespread among fungi and include species from the ascomycetes (Trichoderma reesei), basidiomycetes (Fomitopsis palustris) with few anaerobic species.
- Among fungi, soft rot is the best known for producing cellulases, and among them, Trichoderma is the best-studied.
- Other well-known cellulase-producing soft rots are Aspergillus niger, Fusarium oxysporum, Neurospora crassa, etc.
- Besides soft rots, brown rot and white rot fungi are also actively involved in cellulose degradation; however, the mechanism of action of these enzymes are distinctly different.
- The brown rot actively hydrolyzes cellulose during the earlywood decay as they lack exoglucanases. Some of the common examples of these fungi include Poria placenta, Lenzites trabea, Coniophora puteana, and Tyromyces palustris.
- The white rot, in turn, is mostly in lignocelluloses degradation with examples like Phanerochaete chrysosporium, Sporotrichum thermophile, and Trametes versicolor.
- Among the anaerobic cellulolytic fungi, most studied are the Neocallimastix frontalis, Piromyces (Piromonas) communis, and Orpinomyces species.
Cellulolytic Bacteria
- Cellulolytic bacteria often produce cellulases in small amounts, and degradation of cellulose seems to take place by a cluster of multienzyme complexes.
- Most of the bacterial cellulolytic enzymes are reported from Bacillus, Acinetobacter, Cellulomonas, and Clostridium.
- About 90-95% of all bacterial cellulase activity is observed under aerobic conditions by aerobic bacteria. However, the remaining 10% is degraded by a diverse group of bacteria under anaerobic conditions.
- Besides, some of the rumen bacteria are also known to produce cellulases that can degrade the cell wall components.
- Some of the examples include Fibrobacter succinogenes, Ruminococcus albus, Pseudomonas, Proteus, and Staphylococcus.
- Some thermophilic bacteria like Anoxybacillus sp, Geobacillus sp, and Bacteroides also exhibit cellulase activity.
Enzymes involved in the degradation of cellulose
The enzymes involved in the degradation of cellulose are groups as cellulases. There are about five types of cellulases on the basis of the reactions they catalyzed.
1. Endoglucanase
- Endoglucanases are a group of endocellulase that cleave the cellulose molecule at the internal bonds of the non-crystalline surface of the molecule.
- Endoglucanases randomly attack the cellulose chain and splits β-1, 4-glucosidic linkages present within the molecule.
- Endoglucanases function to reduce the length of the cellulose so that the fragments can be acted upon by other enzymes.
2. Exoglucanases
- Exogluconases are a group of exocellulase that hydrolyze the reducing or non-reducing ends of the cellulose chains.
- The major products of the enzymatic action are cellobioses which are further hydrolyzed into monomeric units.
- Exoglucanases act on the smaller tetrasaccharides and disaccharides formed after the action of endoglucanases.
- Exoglucanases include both 1, 4-β-D-glucan glucanohydrolases, liberating D-glucose from β-glucan and cellodextrins, and 1, 4-β-D-glucan cellobiohydrolases that liberate D-cellobiose from β-glucan in a processive manner.
3. Cellobiases
- Cellobiases are enzymes that act on the cellobiose units (disaccharides, trisaccharides, and tetrasaccharides) to form monomeric units.
- Cellobiases are also called β-glucosidases as they form individual glucose units.
4. Oxidative cellulases
- Oxidative cellulases are enzymes that depolymerize cellulose into smaller units by radical reactions.
- Enzymes like cellobiose dehydrogenase catalyze the conversion of varied forms into cellobiose so that it can be acted upon by cellobiases.
5. Cellobiose phosphorylases
- Cellobiose phosphorylases are similar to cellobiases except that the hydrolysis of polymeric units is brought about in the presence of phosphorus rather than water.
Aerobic and Anaerobic degradation of cellulose
1. Aerobic degradation of cellulose
- Aerobic cellulolysis is performed by the synergistic action of three types of enzymatic activities: endoglucanases or 1, 4-β-D-glucan 4-glucanohydrolases, exoglucanases and β-glucosidases or β-D-glucoside glucohydrolases, resulting in the release of D-glucose units from soluble cellodextrins and a variety of glycosides.
- Aerobic cellulases are produced in high concentrations and also act in a sequential manner.
- Aerobic hydrolysis is fairly simple and occurs in sequential steps, each of the steps catalyzed by a different type of cellulase enzyme.
- Endoglucanases attack amorphous regions of cellulose fibers, forming sites for exoglucanases which can then hydrolyze cellobiose units from more crystalline regions of the fibers.
- Finally, β-glucosidases, by hydrolyzing cellobiose, result in the formation of monomeric glucose units.
2. Anaerobic degradation of cellulose
- The mechanism by which cellulases from anaerobic bacteria catalyze the depolymerization of crystalline cellulose is poorly defined; however, it is known that the mechanism is distinctly different from that of aerobic hydrolysis.
- The cellulases of most anaerobic microorganisms are organized into large, multiprotein complexes, called cellulosomes.
- The cellulosomes mediate a close neighborhood between cell and substrate and thus minimize diffusion losses of hydrolytic products.
Factors affecting cellulose degradation
Cellulose degradation in soil or other is influenced by a number of factors, some of which are:
1. Available minerals
- The availability of nutrients and minerals affects the degradation of cellulose as these components are required for the production of biomolecules like cellulases and other proteins.
- The increase in nutrients and minerals increases the rate of cellulose degradation.
2. Temperature
- Cellulose degradation occurs within the temperature range of 0°C, and 65°C as both psychrophilic and thermophilic organisms are capable of hydrolyzing cellulose.
- However, the degradation of cellulose is optimal at the mesophilic temperature range of 25-30°C.
3. Aeration
- The availability of oxygen affects both the rate and the mechanism of hydrolysis as the enzyme involved, and the mode of action differs in aerobic and anaerobic organisms.
- In the presence of oxygen, a sequential process of hydrolysis of cellulose in glucose occurs by three different groups of enzymes.
- Under anaerobic conditions, the cellulolytic enzymes form a multienzyme complex which is comparatively slower.
4. pH
- Cellulose degradation is slightly higher in acidic soil than alkaline or neutral soil.
- Under the acidic condition, fungi are the primary group of organisms involved in cellulose decomposition, whereas bacteria and actinomycetes act as dominant cellulose decomposers in neutral to alkaline conditions.
5. Organic matter
- The presence of organic matter also increases the rate of cellulose degradation as much of the organic matter act as a substrate.
- However, if cellulose is the only component of the matter, the rate of hydrolysis decreases.
- The rate of degradation increases with the addition of a small amount of readily decomposable organic matter as it allows the growth of microorganisms.
6. Lignin
- The presence of lignin decreases the rate of cellulose degradation.
- Lignin is closely related to cellulose, which affects the degradation of cellulose.
Process (Simple Steps) of cellulose degradation
Cellulose degradation occurs in three simple steps;
1. Hydrolysis by endoglucanases
- The first step in the degradation of cellulose is the action of endoglucanases that randomly attack the cellulose fibrils.
- This step results in a decrease in the size of cellulose chains as it degrades the polymer into smaller fragments.
- The enzyme acts internally at random points of the polymer.
2. Hydrolysis by exoglucanases
- Exoglucanases act on the smaller fragments resulting in even smaller units of tetrasaccharides or disaccharides.
- Exoglucanases act on the reducing end of the fragments to form either dimeric units or cellobiose.
3. Hydrolysis by β-glucosidase
- β-glucosidase or cellobiose act on the dimeric units of glucose of cellobiose to form monomeric units, glucose.
- This is the final step of cellulose degradation that results in the formation of free individual units of the glucose molecule.
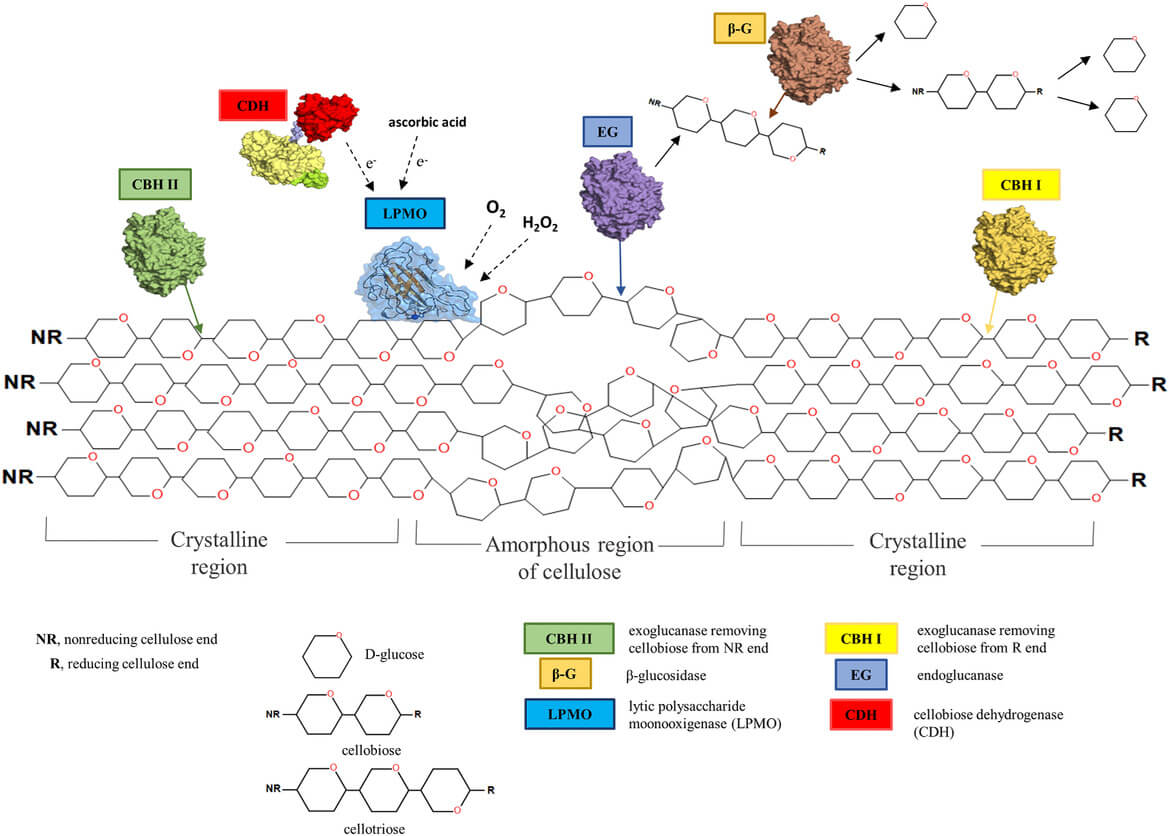
Figure: Scheme of the enzymatic degradation of cellulose chain via synergistic interaction of cellulases (endoglucanase, exoglucanase, and β‐glucosidase) and LPMO (AA9 or 10) enzymes. Image Source: https://doi.org/10.1002/elsc.201800039.
Mechanisms of microbial degradation of cellulose
- Two well-studied mechanisms are utilized by cellulolytic microorganisms to degrade the cellulose present and a third less well studied oxidative mechanism is known to be used by brown-rot fungi.
- Both of the well-studied mechanisms of cellulose degradation occurs by the enzymatic action of cellulases to breakdown β-1, 4 linkages; however, the ways by which the cellulases are presented to the environment are quite different.
- Many studied aerobic microorganisms use the free cellulase mechanism to digest cellulose although brown rot fungi appear to use a different oxidative mechanism for degrading cellulose.
A. Hydrolytic Mechanism of cellulose degradation
- In glycosyl hydrolases, enzymatic hydrolysis of the glycosidic bond usually takes place via general acid/base catalysis, which requires two critical residues: a proton donor (HA) and a nucleophile/base (B-).
- This catalytic activity is provided by two aspartic- or glutamic acid residues.
- Mechanistically, the reactions catalyzed by all cellulases are known to involve general acid-base catalysis by a carboxylate pair at the enzyme active site, even if they are different in structure.
- One of the residues acts as a general acid and protonates the oxygen of the o-glycosidic bond, while the other residue acts as a nucleophile.
- On the basis of the distance between the two carboxylic groups, either inverting (10 Å distances) or retaining (5 Å-distances) mechanisms are observed in cellulases.
1. Inverting mechanism
- In the case of inverting cellulase mechanism, two enzyme residues, typically carboxylate residues, act as acid and base.
- The inverting mechanism is brought about by the attack of a water molecule on the C1 carbon of the glucose ring in an Sn2 type displacement reaction, resulting in inversion of the configuration at the anomeric carbon C1.
2. Retaining mechanism
- In the case of retaining cellulase mechanism, hydrolysis occurs in a two-step mechanism, with each step involving inversion. As in inversion, two enzyme residues are involved where one acts as a nucleophile while the other acts as an acid or a base.
- In the first step, the nucleophile attacks the anomeric center, resulting in deprotonation. The deprotonated carboxylate then acts as a base in the next step that assists nucleophilic water in forming the hydrolyzed product.
3. Glycosidase mechanism
- Recently, a fundamentally different glycosidase mechanism has been discovered for NAD+ and divalent metal ion-dependent GH4 glycosidases.
- In this case, hydride abstraction at C3 generates a ketone, followed by deprotonation of C2 accompanied by acid-catalyzed elimination of the glycosidic oxygen and formation of a 1, 2-unsaturated intermediate.
- This α-β-unsaturated species undergoes a base-catalyzed attack by water to generate a 3-keto derivative, which is then reduced by NADH to complete the reaction cycle.
Example of Hydrolytic Mechanism
- The hydrolytic mechanism is observed in most of the aerobic and anaerobic microorganisms including Bacillus, Acinetobacter, Cellulomonas, Clostridium, Aspergillus niger, Fusarium oxysporum, Neurospora crassa, and Trichoderma reesei.
B. Oxidative Mechanism of cellulose degradation
- Even though most aerobic bacteria degrade cellulose by the synergistic action of different cellulases, some cell-free cellulolytic fungal culture filtrates degrade cellulose faster in an oxygen atmosphere than under anaerobic conditions.
- In this case, the enzyme cellobiose dehydrogenase (CDH) is found to play an important role.
- CDH catalyzes in a ping-pong type reaction in the oxidation of cellobiose (the main product of cellulase action) to cellobionolactone under the reduction of various electron acceptors such as quinones, chelated Fe(III), O2 (producing hydrogen peroxide), and phenoxyl radicals.
- Besides, the cellobiose dehydrogenase also has further roles in cellulose degradation.
- CDH oxidizes free ends created by endo-acting cellulases and prevents re-condensation of the cellulose chain.
- Product inhibition is prevented by the removal of cellobiose, as high concentrations of this disaccharide inhibit many cellulases.
- CDH can produce Fe2+ and H2O2 by the reduction of Fe3+ and O2. Together they form hydroxyl radicals in a Fenton-type reaction, which depolymerizes or modifies the cellulose.
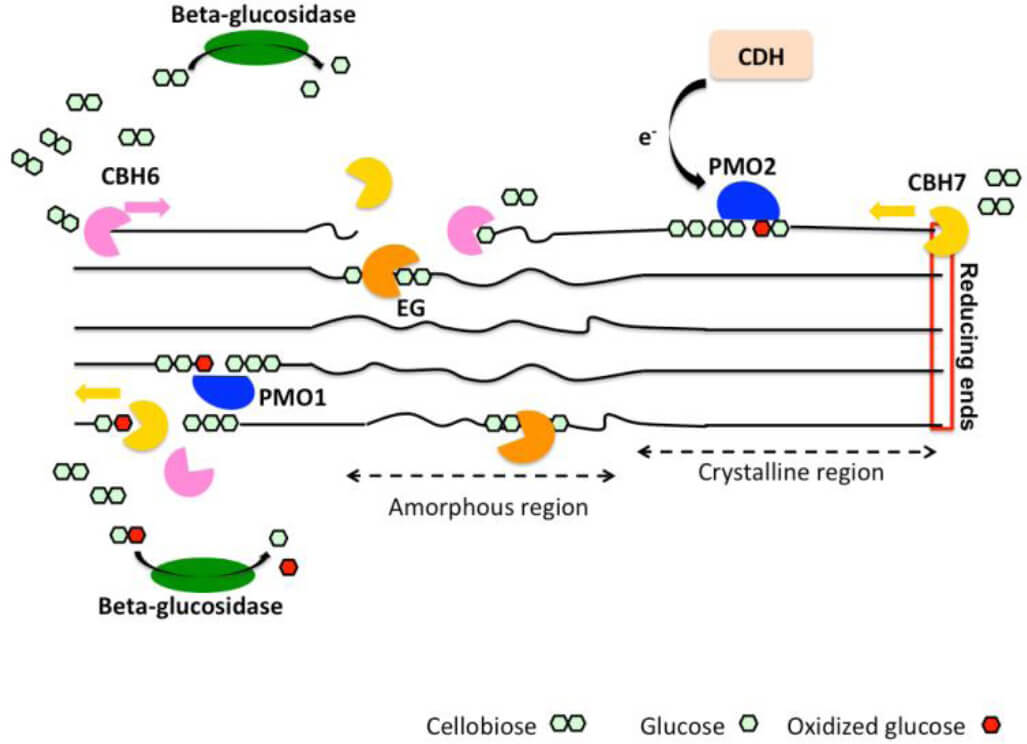
Figure: Oxidative Mechanism of cellulose degradation. Image Source: https://doi.org/10.5936/csbj.201209015.
Example of Oxidative Mechanism
- Examples of oxidative mechanisms can be observed in fungal species like Phanerochaete chrysosporium, Sporotrichum thermophile, Poria placenta, Lenzites trabea, etc.
References
- Gupta, R., Mehta, G., Deswal, D., Sharma, S., Jain, K. K., Kuhad, R. C., & Singh, A. (2013). Cellulases and Their Biotechnological Applications. Biotechnology for Environmental Management and Resource Recovery, 89–106.DOI:10.1007/978-81-322-0876-1_6
- P. Gautam, P. S. Bundela, A. K. Pandey, Jamaluddin, M. K. Awasthi, S. Sarsaiya, “Diversity of Cellulolytic Microbes and the Biodegradation of Municipal Solid Waste by a Potential Strain”, International Journal of Microbiology, vol. 2012, Article ID 325907, 12 pages, 2012. https://doi.org/10.1155/2012/325907
- Suriya, S. Bharathiraja, P. Manivasagan, S.-K. Kim. Chapter Four – Enzymes From Rare Actinobacterial Strains. Advances in Food and Nutrition Research. Volume 79, 2016. https://doi.org/10.1016/bs.afnr.2016.08.002.
- B. Wilson. Cellulases. Encyclopedia of Microbiology (Third Edition). 2009. https://doi.org/10.1016/B978-012373944-5.00138-3.
- Wilson, D. B. (2009). Evidence for a novel mechanism of microbial cellulose degradation. Cellulose. 16(4), 723–727.DOI:10.1007/s10570-009-9326-9
- Barbosa, F.C., Silvello, M.A. & Goldbeck, R. Cellulase and oxidative enzymes: new approaches, challenges and perspectives on cellulose degradation for bioethanol production. Biotechnol Lett42, 875–884 (2020). https://doi.org/10.1007/s10529-020-02875-4
- Ogundero, V.W. (1983). Factors affecting growth and cellulose hydrolysis by the thermotolerant Aspergillus nidulans from composts. Acta Biotechnol., 3: 65-72. https://doi.org/10.1002/abio.370030116
- Kruså, M., Henriksson, G., Johansson, G., Reitberger, T., & Lennholm, H. (2005). Oxidative cellulose degradation by cellobiose dehydrogenase from Phanerochaete chrysosporium: A model compound study. Holzforschung, 59(3).doi:10.1515/hf.2005.043
- Coughlan, M. P. (1991). Mechanisms of cellulose degradation by fungi and bacteria. Animal Feed Science and Technology, 32(1-3), 77–100.DOI:10.1016/0377-8401(91)90012-h
- Schwarz W. H. (2001). The cellulosome and cellulose degradation by anaerobic bacteria. Applied Microbiology and Biotechnology, 56(5-6), 634–649.DOI:10.1007/s002530100710.
Your work is very nice and educative
I just couldn’t get a DOI link to reference it in my study
Hi, You can cite as follows:
Sapkota, A. (2022, September 15). Microbial degradation of cellulose (Enzymes, Steps, Mechanisms). Retrieved from https://microbenotes.com/microbial-degradation-of-cellulose/
Thank youu,