Epistasis is the interaction between two non-allelic genes where the phenotypic expression of one gene is masked or suppressed by the expression of one or more other genes.
Earlier, Mendelian and his coworkers assumed that the traits were governed by the expression of a single gene with two alleles, one of which being dominant over the other. Later, it was discovered that the expression of two or more genes could control the development of certain traits. Thus, Mendelian genetics failed to identify such different kinds of inheritance for which their phenotypic ratios differ from Mendelian ratios (monohybrid-3:1 and dihybrid- 9:3:3:1 in F2 generation).
William Bateson and R.C. Punnett proposed the “Factor Hypothesis” where they explained genetic interaction. This states that certain characters are governed by the expression of two or more genes. Gene interaction occurs as a result of interaction between two or more allelic or non-allelic genes of the same genotype. As a consequence of this, variation in particular phenotypic characters occur.
The expression of one gene relies on the expression (i.e., presence or absence) of another gene, i.e., the expression of genes is not independent of each other. Two types of genetic interactions exist, namely:
- Allelic/ Intra-allelic gene interaction/Intragenic interaction
- Non-allelic /Inter-allelic gene interaction/Intergenic interaction
Intragenic interaction is the genetic interaction between the two alleles of a single gene. However, intergenic interaction is the genetic interaction between two or more genes located in the same or different chromosome(s). Two or more gene products are involved in the outcome of one common characteristic.
Interesting Science Videos
What is Epistasis?
William Bateson first coined the term “epistatic” in 1909 to describe the genetic interaction in which one mutation masks or hides the effects of other mutations. Later, R.A. Fischer used the derived term “epistacy” to describe statistical deviation from the cumulative addition of loci in the phenotypic expression.
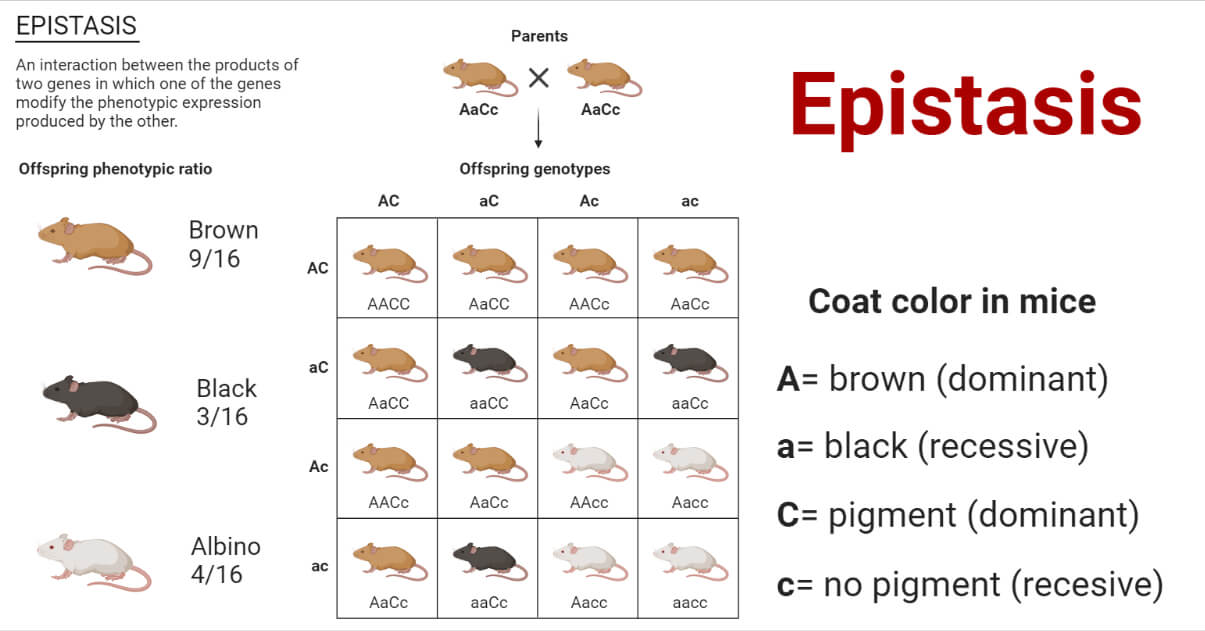
It has also been defined as the deviation from the expected outcome in the form of phenotypic traits when combining mutations. Epistasis can be evident under the following circumstances:
- Two or more loci interact to produce new phenotypes.
- Presence of an allele at one locus masks the effects of alleles at one or more loci.
- The presence of an allele at one locus alters the effects of alleles at one or more loci.
Epistasis occurs at the phenotypic level of organization. The expression of one particular gene not only influences the phenotype but hides the output of other genes interacting together.
Different classes of Epistasis
Epistatic interactions are categorized into different classes based on:
Outcome
Based on the outcome, epistasis is classified as positive and negative epistasis.
If the outcome (as a result of mutation(s)) is better (more fit) than expected in a given genetic background, it’s positive epistasis. In comparison, if the outcome is worse (less fit) than expected, it’s negative epistasis.
Strength and direction of mutation
Magnitude epistasis
It occurs when the magnitude of the effect of mutation changes the genetic background.
Sign epistasis
It occurs when the direction of the effect of mutation changes the genetic background.
Number of mutations
If the epistatic interaction embodies two mutations, it’s called pairwise epistasis. Similarly, the involvement of more than two mutations is called higher-order epistasis.
Specificity
Specific epistasis: In specific epistasis, one mutation modulates the phenotypic effects of a few other mutations.
Non-specific epistasis: Here, a mutation influences the effects of a relatively large number of other mutations.
Measurement of interaction
When the genetic interaction is measured in terms of specific genetic background, it is known as background-relative epistasis. However, the average of all sets of genetic backgrounds is called background-averaged epistasis.
Types of Epistasis
Generally, there are six types of epistasis gene interaction. Namely:
- Dominant epistasis
- Dominant inhibitory epistasis
- Recessive epistasis
- Duplicate dominant epistasis
- Duplicate recessive epistasis
- Polymeric epistasis
Dominant Epistasis
- A dominant allele of one gene conceals the phenotypic expression of both recessive and dominant alleles at other loci of other genes.
- It is also known as simple epistasis.
- Example: Fruit color in Summer Squash (Cucurbita pepo)
- It modifies the classical ratio of 9:3:3:1 into 12:3:1 for the F2 hybrid.
- White, yellow, and green are the three different colors of squash fruits.
- The allele for white color is dominant over both yellow and green. Similarly, yellow is dominant over green color.
- If the dominant allele W is present, it masks the phenotypic effect of the yellow and green allele, and thus, the fruit becomes white.
- In the chart above, allele W is dominant to allele w and epistatic to alleles G and g. A cross made between white fruit and yellow fruit produces F1 white fruits. This F1, when allowed to interbreed, resulted in F2 progeny of green, yellow, and white colored fruits in the ratio of 12:3:1.
Dominant Inhibitory Epistasis
- A dominant allele at one location blocks the expression of both dominant and recessive alleles at the second locus.
- It is also known as inhibitory gene interaction.
- Example: Anthocyanin pigmentation in rice
- The standard dihybrid segregation ratio of 9:3:3:1 is changed to 13:3 in the F2 generation.
- In the above figure, a dominant allele, I controls the green color while P controls the purple coloration in rice. A cross between the green and purple plants produced green plants in the F1 generation. Again, in-breeding of F1 progeny resulted in the production of green and purple plants in the ratio of 13:3 in the F2 generation.
- Here, allele I is dominant to i and epistatic to alleles P and p. Thus, the presence of allele I conceals the effects of P and p and produces green color; however, the absence of allele I in iiPP and iiPp produces purple plants.
Recessive Epistasis
- The presence of recessive alleles of one gene hides the phenotypic expression of both dominant and recessive alleles at other loci of other genes.
- It is also known as supplementary epistasis.
- Example: Coat color in Labrador retrievers and grain color in maize
- It modifies the classical ratio 9:3:3:1 into 9:3:4 for the F2 hybrid.
- The above figure illustrates recessive epistasis for grain color in maize. Typically, there are three different colors of grains in maize. Namely: Purple, Red, and White grains. The presence of two dominant alleles (R and P together) leads to the development of the purple color. Similarly, the occurrence of only R as the dominant gene produces red color and white in homozygous conditions.
- Initially, the cross between white and white grains produced purple grains in F1. Moreover, when F1 progenies were crossed with each other, it resulted in white, red, and purple grains in F2 generation in the ratio of 9:3:4.
- Allele r is epistatic to alleles P and p but recessive to R.
Duplicate epistasis
- The presence of a dominant allele at either of two loci conceals the expression of complementary recessive alleles at the two loci.
- It is also known as duplicate gene interaction or duplicate dominant epistasis.
- Examples: Awn character in rice, Seed capsules of Shepherd’s purse (Capsella spp.)
- The normal dihybrid ratio of 9:3:3:1 is modified to 15:1 in the F2 generation.
- Duplicate alleles A and B control the awn character in rice. The presence of one either of them is essential for developing awn character, which becomes asymptomatic when present in a homozygous recessive condition.
- Cross between awned and awnless rice produces awned rice in F1 progeny. In-breeding between F1 progenies resulted in awned and unawned plants in 15:1 ratio in the F2 generation.
- A dominant allele A is epistatic to alleles B and b. Similarly, dominant allele B is epistatic to alleles A and a. The presence of either or both of these dominant alleles is responsible for the awn character in rice. However, the occurrence of the double homozygous recessive gene (aabb) results in awnless in rice.
Duplicate recessive epistasis
- The presence of recessive alleles at either of the two loci masks the expression of dominant alleles in the two loci.
- It is also known as complementary gene action because the two genes function together to produce outcomes.
- Example: Flower color in sweet pea, Lathyrus odoratus.
- The normal dihybrid ratio of 9:3:3:1 is altered to 9:7 in F2 progeny.
- The purple color of the flower in sweet peas is due to the presence of two dominant genes A and B, as represented above. Similarly, whenever these genes are present in recessive form (aabb) or separate individuals (AAbb or aaBB), a white flower is produced.
- The above figure depicts the cross between purple and white flower, which produced purple color in F1 progeny. Likewise, interbreeding of F1 progenies produced purple and white flowers in the ratio of 9:7 in the F2 generation.
- The recessive allele a is epistatic to B and b alleles, and recessive allele b is epistatic to alleles A and a.
Polymeric gene interaction
- The presence of a dominant allele at two or more loci acts synergistically to produce a unique phenotype with greater effects than it would produce when present individually.
- It is also known as duplicate gene interaction with cumulative effect.
- Example: Fruit shape in Summer Squash.
- The normal dihybrid ratio of 9:3:3:1 is modified to 9:6:1 in F2 progeny.
- Three types of fruit shapes can be observed in summer squash, namely disc, spherical and long, in which two dominant genes A and B, control disc shape. Either A or B produces the spherical shape, and the presence of a double recessive gene (aabb) develops long-shaped fruits.
- The above figure portrays the polymeric gene interaction for fruit shape in summer squash in which a cross between disc shape and long shape fruit produced disc shape fruit in the F1 generation, which on interbreeding produced disc shape, spherical and long shape fruit in the ratio of 9:6:1 in the F2 generation.
Implications of epistasis
- Epistasis is important in the comprehensive study of the structure and function of genetic pathways.
- Epistatic interaction has played a crucial role in shaping the molecular evolution of infectious diseases like HIV and Influenza and in the emergence of antibiotic resistance.
- The exploitation of epistasis in the field of oncology helps in drug discovery for cancer treatment.
- Epistatic patterns help to disclose the structure of proteins and their nature of physical interaction and thus contribute to protein design.
- It has wide implications in the health sector, and personalized medicine makes it possible to predict how the pathogen or patient will respond to treatments.
References
- https://byjus.com/neet/what-is-epistasis/
- https://www.biologydiscussion.com/genetics/epistasis-definition-and-types-genetics/67404
- https://biologydictionary.net/epistasis/
- https://bio.libretexts.org/Bookshelves/Introductory_and_General_Biology/Book%3A_General_Biology_(Boundless)/12%3A_Mendel’s_Experiments_and_Heredity/12.03%3A_Laws_of_Inheritance/12.3F%3A_Epistasis
- https://unacademy.com/content/neet-ug/study-material/biology/epistasis/
- https://study.com/academy/lesson/dominant-vs-recessive-epistasis-example-analysis.html
- https://www.sciencedirect.com/topics/computer-science/epistasis
- https://www.annualreviews.org/doi/10.1146/annurev-genom-083118-014857
- https://microbiologynote.com/epistasis/
- https://www.yourarticlelibrary.com/biology/6-most-important-kinds-of-epistasis-biology/6436
- https://www.nature.com/scitable/topicpage/epistasis-gene-interaction-and-phenotype-effects-460/