Chlorophyll is an essential class of pigment molecules that act as a principal photoreceptor in the case of most green plants.
- Chlorophyll is a photosynthetic pigment that is involved in absorbing electromagnetic radiation and aids in the conversion of light energy to chemical energy via the synthesis of organic compounds. The term ‘chlorophyll’ is derived from two Greek words; khloros meaning pale green and phyllon meaning leaves.
- Photosynthetic pigments usually have different colors, and they absorb light of different wavelengths depending on the color.
- Chlorophyll is unique to other pigments in that it absorbs light rays of a particular wavelength that have enough energy to build tissues.
- Chlorophylls in plants are present in all green parts and are localized in the form of disks-like units called thylakoids in the chloroplasts of each cell.
- Chlorophyll mostly absorbs the blue portion of the electromagnetic radiation with some radiation from the red portion. It doesn’t, however, absorbs the green portions and instead reflects it, producing the characteristic green color.
- Even though chlorophyll is most prevalent in green plants, it is found in virtually all photosynthetic organisms ranging from algae to some bacteria. The chlorophyll found in different living beings might be of different types.
- The chlorophyll found in leaves exists in the form of light-harvesting complexes, and its light-absorbing properties are also quite different.
- In the free form, chlorophyll becomes excited by light and quickly releases energy in the form of fluorescence.
- In the intact form in leaves, however, the absorbed energy is passed on to the next molecules with very little fluorescence.
- Chlorophyll thus is an important pigment that is responsible for the absorption of energy from light and its transfer into the chemical form so that it can be utilized by living organisms.
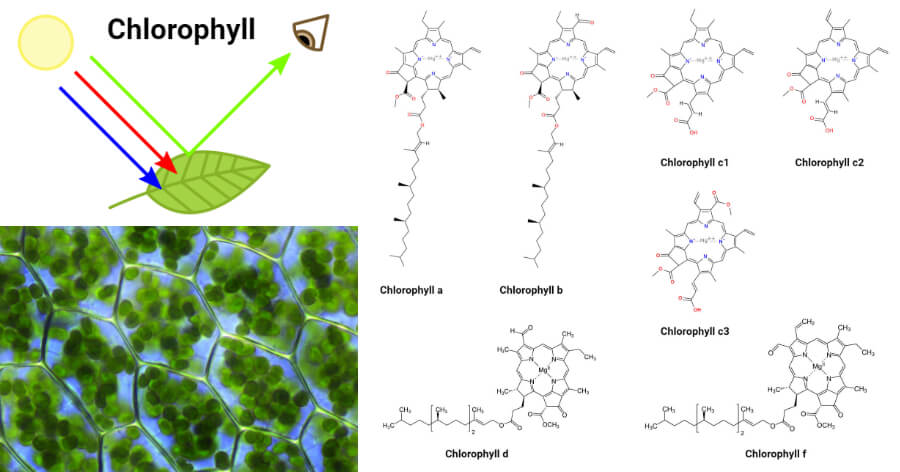
Image Source: Wikipedia.
Interesting Science Videos
Chlorophyll Structure
- The chlorophyll molecule consists of a central metal core surrounded by a nitrogen-containing structure, resulting in a porphyrin ring.
- All the chlorophyll molecules are characterized by the presence of four pyrrole-like rings (thus termed tetrapyrroles) along with an additional fifth ring.
- Besides, a number of side chains are attached to the ring structure, of which an important one is, the side chain of a long hydrocarbon, called phytol ring.
- The molecular formula of chlorophyll is C55H72MgN4O5.
- Chlorophylls are classified as chlorins, which are close relatives of porphyrins like hemoglobin.
- There are different types of chlorophylls that might differ in their chemical structures as they occur in different living organisms.
- Among all different types of chlorophylls, the structure of chlorophyll a is the most studied.
- Structurally, chlorophylls are different from other photosynthetic pigments in that they have networks of alternating single and double bonds which make them quite effective towards photoreception.
- The electrons in the molecules are not localized but remain distributed throughout the bonds, which enable the pigment to absorb light more readily.
Chlorophyll Types
Chlorophyll a
- Chlorophyll a is the most widely distributed type of chlorophyll which is found in all living organisms capable of oxygenic photosynthesis (producing oxygen as a by-product).
- It has also been reported in small quantities in some sulfur bacteria performing anaerobic photosynthesis.
- The pigment absorbs most of the wavelength present within the blue-violet and orange-red part of the light, and reflects the yellow-green light, resulting in the appearance of green color of such parts.
- It is found in most of the photosynthetic organisms belonging to eukaryotes, cyanobacteria, and prochlorophytes where they act as a primary electron donor in the electron transport chain.
- The structure of chlorophyll a is similar to the basic structure of chlorophyll with a chlorin ring where four nitrogen atoms surround the magnesium ion.
- Chlorophyll a is different from other chlorophyll in the type of side-chain attached to the ring. It has electron-donating methyl and ethyl groups at the C-7 and C-8 positions, which distinguished it from chlorophyll b.
- The hydrocarbon tail in the case of chlorophyll a is hydrophobic which enables the binding of the molecule to other hydrophobic proteins in the thylakoid membrane of the chloroplast.
- The biosynthesis of chlorophyll a takes place by the reaction between chlorophyllide a and phytyl diphosphate in the presence of the enzyme chlorophyll synthase.
Chlorophyll b
- Chlorophyll b is the second most abundant chlorophyll in oxygenic photosynthetic organisms. These are distinguished from chlorophyll a in the formyl substitution in the C-7 position of the ring.
- Chlorophyll b is present as a part of the components of the peripheral antenna complexes.
- It is synthesized by the oxidation of the methyl group present in the chlorophyll a to a formyl group.
- The molecular formula of chlorophyll b is C55H70MgN4O6, and it absorbs most of the blue light in the wavelength spectrum.
- Chlorophyll b is found in most land plants around the photosystem II and in the shade-adapted chloroplasts, the ratio of chlorophyll b is more in photosystem II than in photosystem I.
- The concentration of chlorophyll b might increase with the adaptation of the organisms, and with the increase in chlorophyll b, the range of wavelength absorbed by the organisms also increases.
- The process of biosynthesis of chlorophyll b is similar to that of chlorophyll a, and it takes place in the presence of the enzyme chlorophyll synthase.
Chlorophyll c
- Chlorophyll c is a form of chlorophyll that acts as an accessory pigment and is less widely distributed than chlorophyll a and b.
- These pigments are found in the golden-brown eukaryotic algae, marine algae, and dinoflagellates.
- Chlorophyll c produces a blue-green color as it absorbs light in the 447-520nm wavelength regions. The molecular formula of chlorophyll c is C35H28O5N4Mg.
- Structurally, chlorophyll c is different from other chlorophylls in that it has a porphyrin ring structure without an isoprenoid tail or a reduced ring D.
- They have a trans acrylic acid at C-17 (ring D), instead of the propionic acid side chain of chlorophyll a and b, which in all polar chlorophyll c pigments is not esterified to phytol or other aliphatic long-chain alcohol.
- The chlorophyll pigments present in the chlorophyll c form are further divided into chlorophyll c1, chlorophyll c2, and chlorophyll c3. Recently, however, five new chlorophyll c have also been identified.
- Chlorophyll c1 is a common form of chlorophyll c which differs from c2 in the presence of an ethyl group instead of the vinyl group at the C-8 position.
- Chlorophyll c2 is the most common form of chlorophyll c which has a vinyl group at the C-8 position of the molecule.
- Chlorophyll c3 is a polar chlorophyll that is isolated from the haptophyte alga Emiliania huxleyi and the picoplanktonic chrysophyte Pelagococcus subviridis.
Chlorophyll d
- Chlorophyll d is one of the rarer forms of chlorophylls that are found in some species of red algae and cyanobacteria.
- It is mostly found in marine algae, where it acts as an adaptation to suit algae and photosynthetic organisms found in deep water where not much light can penetrate.
- Chlorophyll d absorbs far-red wavelengths beyond the optical range and some wavelengths of the blue-green region.
- Structurally, chlorophyll d is similar to chlorophyll b but differs from chlorophyll a in the presence of a formyl group in ring A of the structure.
- The molecular formula of chlorophyll d is C54H70MgN4O6.
- Chlorophyll d is an important pigment in free-living cyanobacteria that live in light environments acquiring less visible light and enhanced infrared radiation.
- In some cases, chlorophyll d even replaces chlorophyll in its light-harvesting role in the reaction centers of photosynthesis.
Chlorophyll e
- Chlorophyll e is a rare form of chlorophyll that is found in some golden algae, primarily two species, Tribonema bombycinum, and Vaucheria hamata.
- Chlorophyll e is found to be similar to bacteriochlorophylls that are distributed in cyanobacteria. It acts as an accessory pigment in different photosynthetic organisms.
- Much is not known about chlorophyll e as it was discovered pretty recently.
- The structure and molecular formula of chlorophyll e are not yet known.
Chlorophyll f
- Chlorophyll f is the newest form of chlorophyll which was discovered from stromatolites in 2010.
- The exact function of chlorophyll f in photosynthesis is not clear yet, but some evidence of it acting as accessory pigments can be found.
- Chlorophyll f is different from other chlorophylls in that it can absorb infrared light present in the red region than other chloroplasts.
- It has, however, been observed that the production of these far-red chlorophylls improves the efficiency of oxygenic photosynthesis.
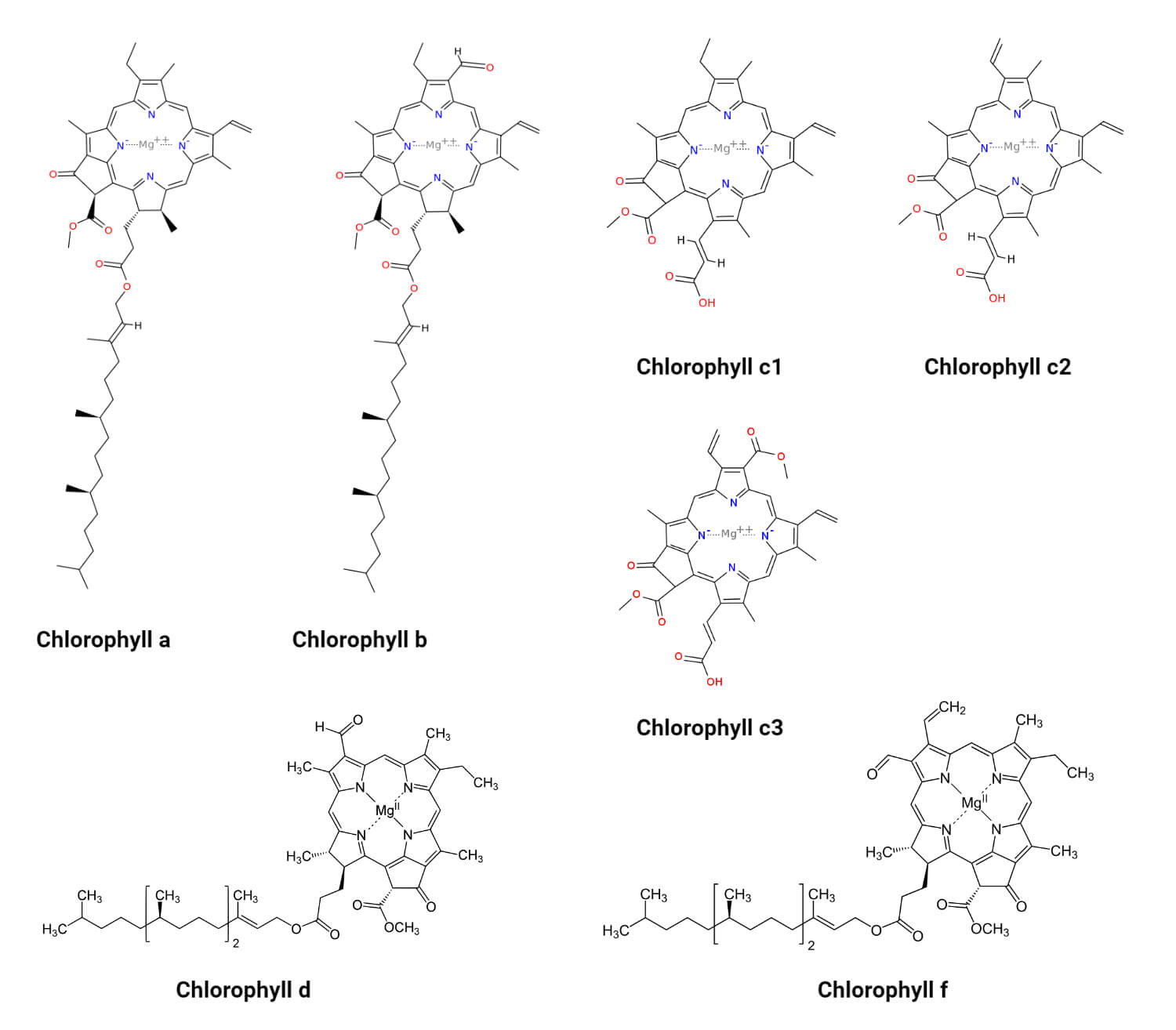
Figure: Structure of different types of Chlorophyll. Image Source: David Richfield (Chlorophyll a,b,c1,c2,c3) and Yikrazuul (Chlorophyll d,f).
Chlorophyll Biosynthesis
- The biosynthesis of chlorophyll in higher plants takes place in the plastids as all the enzymes involved in the process are nuclear-encoded and post-translationally imported to chloroplasts.
- Chlorophyll biosynthesis takes place along with the production of other pigments like carotenoids and pigment-binding proteins.
- The overall process of chlorophyll biosynthesis can take place in the form of two pathways; light-independent pathway and light-dependent pathway.
- The biosynthesis begins with the light-independent pathway, which occurs in the absence of sunlight followed by a light-dependent pathway which occurs as the last-steps of chlorophyll biosynthesis.
- In the case of bacterial chlorophyll, the biosynthesis occurs only via the light-independent pathway, whereas the light-dependent pathway is the only pathway in the case of angiosperms.
- Both of the pathways occur only in the case of algae, cyanobacteria, mosses, and ferns.
- The ability of different organisms to synthesize chlorophyll in the absence of light is due to the production of specific light-independent enzymes.
- A key regulatory step of chlorophyll biosynthesis in green plants is the trans-reduction of ring D of protochlorophyllide to form chlorophyllide.
- The conversion is catalyzed in the presence of nuclear-encoded chloroplast enzyme, protochlorophyllide oxidoreductase.
- The enzyme produced by angiosperm requires light energy for catalysis, which results in the dependence on light for chlorophyll biosynthesis.
- In the case of gymnosperms, algae, and some other plants, an enzyme that reduces protochlorophyllide irrespective of light is produced. This enables the organisms to produce chlorophyll in the dark.
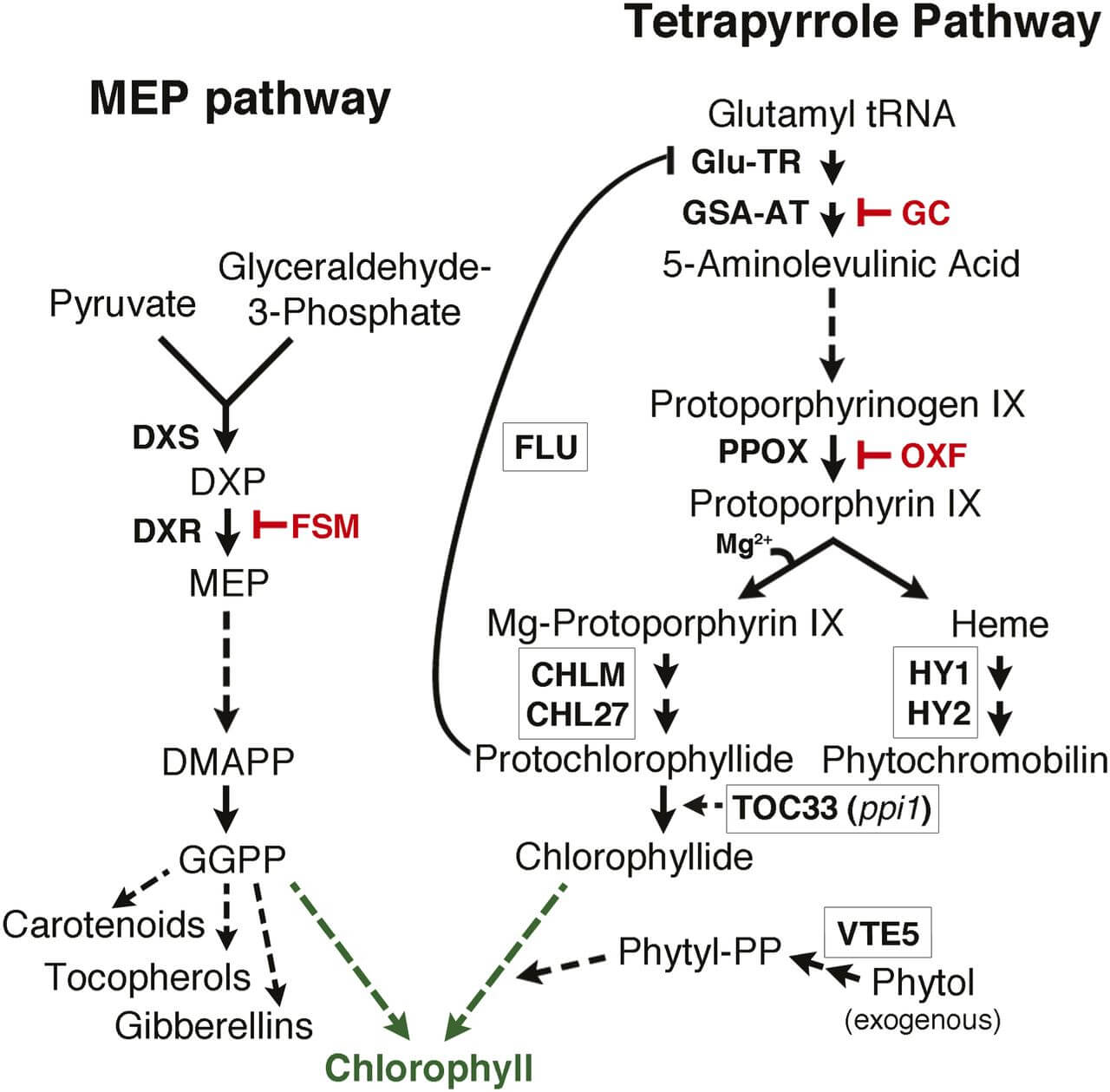
Figure: The MEP and Tetrapyrrole Pathways Collaborate in Chlorophyll Biosynthesis. Image Source: Plant Cell.
The overall process of chlorophyll biosynthesis in higher plants can be explained in the following steps:
1. Ligation of glutamate to tRNA
- The first step of chlorophyll biosynthesis is the ligation of glutamate to tRNA in the presence of glutamyl-tRNA synthetase.
- In the case of eukaryotic cells, two different kinds of glutamyl-tRNA synthetase are present, one in the chloroplast and the other in the cytosol.
2. Reduction of glutamyl-tRNA
- Glutamyl-tRNA reductase is the second enzyme of the biosynthesis pathway that catalyzes the reduction of the activated α-carbonyl group of glutamyl-tRNA.
- This step takes place in the presence of NADPH and releases glutamate -1-semialdehyde.
- For the reaction to take place, pyridine nucleotides are required.
3. Transfer of amino group
- Glutamate 1-semialdehyde is then converted into 5-aminolevulinate in the presence of glutamate 1-semialdehyde enzyme.
- The enzyme is an aminomutase that transfers the amino group from the C-2 of the substrate to the neighboring carbon atom (C-5).
4. Condensation of two 5-aminolevulinate
- The next step is the condensation of two 5-aminolevulinate molecules in the presence of 5-aminolevulinic dehydrogenase, also known as porphobilinogen synthases, to form porphobilinogen.
5. Formation of coproporphyrinogen
- Four molecules of porphobilinogen then combine to form the linear tetrapyrrole, hydroxymethylbilane in the presence of the enzyme porphobilinogen deaminase.
- The hydroxymethylbilane is then acted upon by uroporphyrinogen decarboxylase to produce coproporphyrinogen III.
6. Decarboxylation of coproporphyrinogen
- The next step is the oxidative decarboxylation of the propionate side chains on the ring A and B of coproporphyrinogen molecule to yield protoporphyrinogen IX.
- Protoporphyrinogen oxidase then catalyzes the oxygen-dependent aromatization of protoporphyrinogen into protoporphyrin.
7. Insertion of Mg ions
- The protoporphyrin is then converted into Mg-protoporphyrin by the insertion of Mg2+ ions in the presence of the Mg-chelatase enzyme.
- This process is a two-step reaction, both of which are ATP-dependent.
8. Formation of isocyclic ring
- In the next step, the Mg-protoporphyrin is converted into protochlorophyllide. The reaction is catalyzed by M-protoporphyrin cyclase which results in the formation of an isocyclic ring E of the Mg-protoporphyrins.
- This step can occur both aerobically and anaerobically. The aerobic pathway is common in plants and algae, whereas the anaerobic pathway occurs in bacteria.
9. Conversion of protochlorophyllide into chlorophyllide
- The conversion of protochlorophyllide into chlorophyllide is the only light-requiring step of the pathway.
- The step is catalyzed by protochlorophyllide oxidoreductase, which requires light for the addition of C17 and C18 on the ring D.
10. Formation of chlorophyll
- The final step of the chlorophyll biosynthesis pathway is the conversion of chlorophyllide into chlorophyll in the presence of chlorophyll synthetase enzyme.
- The formation of different types of chlorophyll depends on the presence of different enzymes that catalyze the conversion.
Liquid chlorophyll
- Liquid chlorophyll is the liquefied extract of chlorophyll collected from different green plants.
- Liquid chlorophyll is either collected from green plants like wheatgrass by juicing or can be taken as supplements.
- The use of liquid chlorophyll has increased over the years due to its health benefits.
- The liquid chlorophyll obtained as supplements are actually called chlorophyllin, and they contain copper as the central metal in the place of magnesium.
- The properties of chlorophyllin are similar to that of chlorophyll, and it has similar health benefits.
- Liquid chlorophyll is in the liposoluble state, and it doesn’t dilute in water. It is minimally absorbed, which is why chlorophyll extracted from green plants isn’t absorbed properly.
- Due to the decreased absorption of magnesium chlorophyll, a bioavailable form of chlorophyll is used as a supplement.
- The chlorophyllin used as a supplement has about 2000 times more antioxidant capacity when compared to berries.
- Liquid chlorophyll is taken by mixing it with fresh water. In some cases, the liquid chlorophyll is flavored, which increases its freshness.
- Commercial liquid chlorophyll comes in different concentrations which are used for different purposes. High-quality chlorophyll doesn’t have any preservatives.
- Liquid chlorophyll has numerous health benefits ranging from fresh breath to an increase in blood cells.
Applications/Uses and Health Benefits
Applications/Uses
- Different products produced as derivatives of chlorophylls are used for coloring purposes in industries.
- The pigment is also used in various pharmaceutical and cosmetic products as a wound healing or coloring agent.
- Chlorophyll also acts as a biomordant to enhance the dyeing process of textile products.
- Chlorophyll and chlorophyll derivatives can also be used as coating or protective agents due to their ability to form laminar films with either hydrophilic or hydrophobic properties.
- Chlorophyll is also used as chelating agents due to its complex molecular structure.
- Due to the unique structure of chlorophyll molecules, these act as oxidation-reduction catalysis in both chemical and photosensitized reactions.
- Some amount of chlorophyll is also added to sun protection creams due to its ability to provide protection against polychromatic radiation.
- A derived form of chlorophyll, chlorophyllin, is taken as a health supplement by immune-compromised individuals.
Health Benefits
- Chlorophyllin is known to reduce inflammation of the skin and prevent bacterial growth on skin wounds.
- Chlorophyll also increased the production of blood cells (both RBCs and WBCs) while also improving the quality of these cells.
- Based on different studies, chlorophyll indicates anti-carcinogenic properties which help in the reduction of risks of cancer.
- Chlorophyll has the ability to absorb toxins which helps in the detoxification of the digestive system.
- The use of chlorophyll is also associated with weight loss; however, the research on this topic is quite limited.
- Chlorophyll acts as an internal deodorant that helps with odors from bad breath, sweat, urine, and food odors.
- Chlorophyll helps in digestion by acting as a regulator of intestinal fermentation. It reduces the production of gas in the intestine and even has an antibacterial effect.
Side effects of Chlorophyll
Even though chlorophyll or chlorophyllin are not known to be toxic, it has some possible side effects.
- In some cases, chlorophyll might cause digestive problems in some individuals.
- Diarrhea along with, green, yellow or black stool can be produced which might be mistaken for gastrointestinal bleeding.
- While using topically on the skin surface, itching, or irritation might be observed. A patch test should be done before use.
- When used in large amounts, liquid chlorophyll might induce allergic reactions.
References
- Miyashita, Hideaki & Ikemoto, Hisato & Kurano, Norihide & Adachi, Kyoko & Chihara, Mitsuo & Miyachi, Shigetoh. (1996). Chlorophyll d as a major pigment. Nature. 383. 402-402. 10.1038/383402a0.
- Zapata M., Garrido J.L., Jeffrey S.W. (2006) Chlorophyll c Pigments: Current Status. In: Grimm B., Porra R.J., Rüdiger W., Scheer H. (eds) Chlorophylls and Bacteriochlorophylls. Advances in Photosynthesis and Respiration, vol 25. Springer, Dordrecht. https://doi.org/10.1007/1-4020-4516-6_3
- Xu, H et al. “Chlorophyll b can serve as the major pigment in functional photosystem II complexes of cyanobacteria.” Proceedings of the National Academy of Sciences of the United States of America 98,24 (2001): 14168-73. doi:10.1073/pnas.251530298
- Eggink, L L et al. “The role of chlorophyll b in photosynthesis: hypothesis.” BMC plant biology 1 (2001): 2. doi:10.1186/1471-2229-1-2
- Tanaka, A et al. “Chlorophyll a oxygenase (CAO) is involved in chlorophyll b formation from chlorophyll a.” Proceedings of the National Academy of Sciences of the United States of America 95,21 (1998): 12719-23. doi:10.1073/pnas.95.21.12719
- Hoober, J Kenneth et al. “Chlorophylls, ligands and assembly of light-harvesting complexes in chloroplasts.” Photosynthesis research 94,2-3 (2007): 387-400. doi:10.1007/s11120-007-9181-1
- National Center for Biotechnology Information. PubChem Compound Summary for CID 16070025, Chlorophyll d. https://pubchem.ncbi.nlm.nih.gov/compound/Chlorophyll-d. Accessed Dec. 4, 2020.
- Suzuki, Jon & Bauer, Carl. (1992). Light-Independent Chlorophyll Biosynthesis: Involvement of the Chloroplast Gene chlL (frxC). The Plant cell. 4. 929-40. 10.1105/tpc.4.8.929.
- Rüdiger W., Oster U., Schoch S., Klement H., Helfrich M. (1998) The Last Steps of Chlorophyll Biosynthesis. In: Garab G. (eds) Photosynthesis: Mechanisms and Effects. Springer, Dordrecht. https://doi.org/10.1007/978-94-011-3953-3_749
- Rüdiger W. (2006) Biosynthesis of Chlorophylls a and b: The Last Steps. In: Grimm B., Porra R.J., Rüdiger W., Scheer H. (eds) Chlorophylls and Bacteriochlorophylls. Advances in Photosynthesis and Respiration, vol 25. Springer, Dordrecht. https://doi.org/10.1007/1-4020-4516-6_14
- Tripathy B.C., Pattanayak G.K. (2012) Chlorophyll Biosynthesis in Higher Plants. In: Eaton-Rye J., Tripathy B., Sharkey T. (eds) Photosynthesis. Advances in Photosynthesis and Respiration, vol 34. Springer, Dordrecht. https://doi.org/10.1007/978-94-007-1579-0_3
- John C. Kephart. “Chlorophyll Derivatives: Their Chemistry, Commercial Preparation, and Uses.” Economic Botany, vol. 9, no. 1, 1955, pp. 3–38. JSTOR.
- Schertz F.M (1927). Commercial Applications of Chlorophyll Derivatives. Eng. Chem.1927, 19, 10, 1152–1153
- Trinugroho, J.P., Bečková, M., Shao, S. et al.Chlorophyll f synthesis by a super-rogue photosystem II complex. Plants 6, 238–244 (2020). https://doi.org/10.1038/s41477-020-0616-4
- Cherepanov D.A et al. Evidence that chlorophyll f functions solely as an antenna pigment in far-red-light photosystem I from Fischerella thermalis PCC 7521. Biochimica et Biophysica Acta (BBA). 2020, https://doi.org/10.1016/j.bbabio.2020.148184.
- Anthony W.D. Larkum, Michael Kühl. Chlorophyll d: the puzzle resolved. Trends in Plant Science. Volume 10. https://doi.org/10.1016/j.tplants.2005.06.005.
- https://www.healthline.com/health/liquid-chlorophyll-benefits-risks