Glycogenolysis is the breakdown of complex carbohydrate glycogen into glucose-1-phosphate, which may then be changed into glucose-6-phosphate and enter the glycolytic pathway to make ATP.
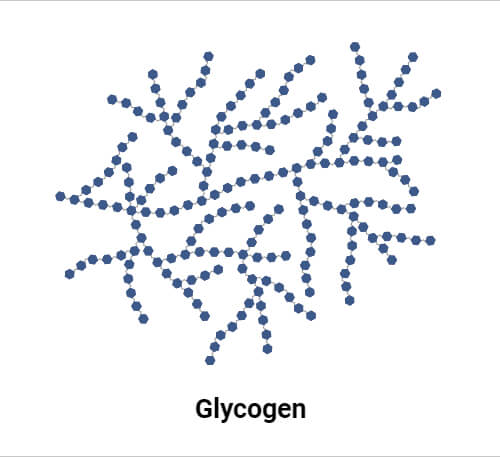
When someone is fasting or when there is a higher need for energy, this procedure is essential to preserving normal blood glucose levels. In this essay, we’ll look at the process, phases involving chemical reactions, use, control, clinical importance, pathology, and summary of glycogenolysis.
Interesting Science Videos
Glycogenolysis Location
Glycogenolysis takes place in the cytoplasm of cells in the muscle, liver, and adipose tissue.
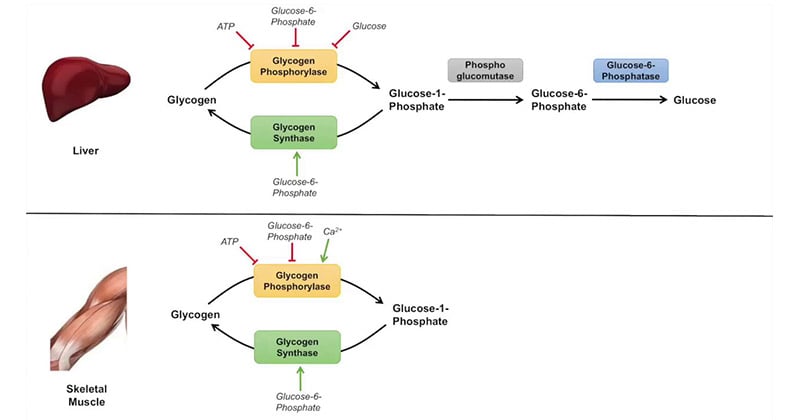
Glycogenolysis Steps and Mechanism
Glycogenolysis occurs mainly in the liver and muscles. It is initiated by the activation of glycogen phosphorylase, an enzyme that catalyzes the removal of glucose residues from the glycogen molecule. The process involves the following steps:
1. Glycogen Phosphorylase Activation
- The enzyme is activated by the hormone glucagon in the liver and by epinephrine in both the liver and muscles.
- Glucagon and epinephrine bind to their respective receptors on the cell surface, triggering a signaling cascade that activates protein kinase A (PKA).
- PKA then phosphorylates and activates phosphorylase kinase, which in turn phosphorylates and activates glycogen phosphorylase.
- Chemical Reaction: Glucagon/epinephrine + Receptor → Signaling cascade → PKA activation → Phosphorylase kinase activation → Glycogen phosphorylase activation
2. Glycogen Phosphorylase Action
- Activated glycogen phosphorylase acts on the α-1,4-glycosidic linkages between glucose molecules in the glycogen polymer.
- It removes glucose residues one by one from the non-reducing ends of the glycogen chain, releasing glucose-1-phosphate.
- Chemical Reaction: Glycogen + Phosphorylase → Glycogen phosphorylase action (Glucose)_n-1 + Phosphate → Glucose-1-phosphate
3. Branching Enzyme Action
- As the glycogen chain is degraded, the branching enzyme (amylo-(1,4→1,6)-transglycosylase) comes into play.
- It moves a block of 3 to 4 glucose units from the main chain to a branch point, generating a new non-reducing end and allowing further glycogen phosphorylase action.
- Chemical Reaction: Glycogen + Branching enzyme → Branching enzyme action (Glucose)_n-1 + (Glucose)_m → (Glucose)_n-1-m + (Glucose)_m+1
4. Debranching Enzyme Action
- Once the glycogen chain is shortened to about four glucose units from a branch point, a debranching enzyme (glycogen-debranching enzyme) hydrolyzes the α-1,6-glycosidic bond, releasing a free glucose molecule.
- Chemical Reaction: Glycogen + Debranching enzyme → Debranching enzyme action (Glucose)_n-1-m + (Glucose)_m+1 → (Glucose)_n-m + Glucose
The activation of glycogen phosphorylase, its impact on the glycogen molecule, the transport of glucose units by the branching enzyme, and the subsequent hydrolysis of branch sites by the debranching enzymes are all components of these processes. When they work in concert, glycogen is broken down into glucose-1-phosphate and free glucose, which may then be used to make ATP.
Functions of Glycogenolysis
The primary purpose of glycogenolysis is to offer a quick supply of glucose for the creation of energy. Glycogen stored in the liver can be broken down to maintain blood glucose homeostasis after fasting or prolonged exercise when blood glucose levels drop. Many organs, including the brain and red blood cells, which strongly rely on glucose as an energy source, can use the glucose produced during glycogenolysis.
Glucose Production
- Glycogenolysis is the primary pathway for glucose production in the body.
- It allows for the breakdown of glycogen stored in the liver and muscles into glucose-1-phosphate, which can be converted to glucose-6-phosphate and further processed in the glycolytic pathway.
- The resulting glucose can be released into the bloodstream to be utilized by various tissues, particularly the brain and red blood cells, which heavily rely on glucose as their main energy source.
Maintenance of Blood Glucose Levels
- Glycogenolysis plays a crucial role in maintaining normal blood glucose levels, especially during fasting or prolonged exercise when glucose demand is high.
- By breaking down stored glycogen, the liver can release glucose into the bloodstream, preventing hypoglycemia (low blood sugar).
- This ensures a constant supply of glucose to vital organs and tissues, allowing them to function properly even in the absence of dietary glucose intake.
Energy Production
- Adenosine triphosphate (ATP), the body’s primary source of energy, is created in cells when glucose from glycogenolysis is metabolized.
- Muscle contraction, active transport, the synthesis of biomolecules, and cellular homeostasis are just a few of the actions that may be carried out by cells with the help of ATP.
Immediate Energy Source during Exercise
- Muscle glycogen acts as a direct source of glucose for energy synthesis inside the muscles themselves during vigorous physical exercise.
- When the oxygen supply is constrained during exercise, glycolysis plays a critical role in supplying the energy required to maintain muscular contractions.
- This enables muscles to continue working without simply depending on aerobic metabolism, which requires oxygen.
Storage and Redistribution of Glucose
- In the liver and muscles, glycogen serves as a rapidly accessible type of glucose storage.
- In times of heightened energy demand or glucose shortage, it acts as a reserve to guarantee a steady supply of glucose.
- Excess glucose is turned into glycogen and stored in these tissues when blood glucose levels are high, as they are after eating.
- Glycogen is broken down by the process of glycogenolysis to produce glucose when blood glucose levels drop or energy requirements rise.
Glycogenolysis Regulations
To provide a sufficient supply of glucose when required, glycolysis is strictly controlled. In this regulation, hormonal control is important.
- When blood glucose levels are low, the pancreas releases glucagon, which starts the liver’s glycogenolysis process.
- When under stress or when exercising, the adrenal glands produce epinephrine, which accelerates the breakdown of glycogen in the muscles and liver. In addition to hormonal control, intracellular concentrations of calcium ions, ATP, and glucose affect glycogenolysis.
- Low concentrations of these molecules encourage the activation of glycogenolysis, whereas high concentrations of glucose and ATP block it.
- The release of calcium ions causes muscle contraction and subsequent glycogen breakdown, which is another function of calcium ions in muscle glycogenolysis.
Hormonal Regulation
1. Glucagon
- A key factor in the activation of glycogenolysis is glucagon, which is secreted by the pancreas in response to low blood glucose levels.
- A signaling cascade that results in the activation of glycogen phosphorylase and the subsequent degradation of glycogen is started when glucagon attaches to its receptors on liver cells.
- Glucagon primarily increases the release of glucose into the circulation to maintain blood glucose levels by stimulating glycogenolysis in the liver.
2. Epinephrine
- During times of stress or physical exertion, the adrenal glands release epinephrine, often known as adrenaline.
- It causes glycogenolysis to begin by acting on both liver and muscle cells. These cells’ receptors for epinephrine attach to them, starting a signaling cascade that activates protein kinase A (PKA).
- Glycogen is produced when PKA phosphorylates and activates glycogen phosphorylase, leading to glycogen breakdown in the liver and muscles.
3. Insulin
- Insulin, released by the pancreas in response to high blood glucose levels, has an inhibitory effect on glycogenolysis.
- Insulin promotes glucose uptake and storage, primarily as glycogen, in liver and muscle cells.
- It inhibits glycogenolysis by dephosphorylating and inactivating glycogen phosphorylase, preventing glycogen breakdown and glucose release.
Enzymatic Regulation
1. Glycogen Phosphorylase
- Phosphorylation and dephosphorylation control the activity of glycogen phosphorylase, the main enzyme involved in the breakdown of glycogen.
- The enzyme is phosphorylated to activate it, and dephosphorylation to inhibit it. Protein kinase A (PKA), which phosphorylates and activates glycogen phosphorylase, is activated by hormones like glucagon and adrenaline through their respective signaling routes.
- Glycogen phosphorylase is dephosphorylated and rendered inactive by protein phosphatase 1 (PP1), which is activated by insulin signaling to stop glycogen breakdown.
2. Glycogen Synthase
- In contrast to glycogen phosphorylase, glycogen synthase is the enzyme in charge of producing glycogen.
- Glycogen synthase is blocked while glycogen phosphorylase is active, inhibiting the simultaneous breakdown and synthesis of glycogen.
Substrate Availability
Glycogenolysis regulation can also be affected by the availability of glucose-6-phosphate, the direct byproduct of glycogenolysis. High concentrations of glucose-6-phosphate block glycogen phosphorylase and slow down the breakdown of glycogen, showing that glucose is already being used for storing or generating energy. Hormonal signals, enzyme changes, and substrate accessibility all play a part in the closely regulated process of glycogenolysis regulation. These control systems make sure that glycogenolysis happens when glucose is required, such as during a fast or physical activity, and that it is prevented when there is enough glucose in the blood to fulfill cellular energy requirements.
Clinical Significance of Glycogenolysis
Glycogenolysis plays a crucial role in maintaining blood glucose levels, and its dysregulation can lead to various clinical conditions. Here are a few examples:
1. Glycogen Storage Diseases
- One type of hereditary illness is known as a glycogen storage disease, which is characterized by a lack of certain glycogen metabolism-related enzymes.
- For example, inadequate levels of glycogen phosphorylase or debranching enzymes might hamper glycogenolysis, causing aberrant glycogen buildup and organ failure.
2. Diabetes
- Glycogenolysis may be improperly controlled in people with diabetes, which results in aberrant glucose synthesis and high blood glucose levels.
- Unchecked glycogenolysis can result in hyperglycemia in type 1 diabetes, which lacks the ability to produce insulin.
3. Exercise and Sports Performance
- Improving athletic performance requires an understanding of how glycogenolysis is controlled.
- In order to maximize muscle glycogen reserves and extend the time before glycogen depletion sets in, athletes frequently follow dietary and training regimens.
Glycogenolysis Pathology
The pathogenic diseases that might impact glycogenolysis are many. For instance, liver conditions like cirrhosis and hepatitis can impede glycogenolysis and glucose generation by interfering with glycogen metabolism. Glycogenolysis can be impacted by a number of hormonal conditions, including Cushing’s disease and growth hormone insufficiency.
Conclusion
A crucial metabolic process called glycolysis produces glucose for energy generation during fasting or other times when the body needs more energy. Glycogen is broken down into glucose-1-phosphate by the activation of glycogen phosphorylase.
Hormones, glucose, ATP, and calcium ions control glycolysis. Diabetes and glycogen storage disorders are two examples of clinical illnesses that can be exacerbated by this mechanism’ dysregulation.
Maintaining normal blood glucose levels and enhancing athletic performance need an understanding of the mechanics and management of glycogenolysis.
References
- David Hames and Nigel Hooper (2005). Biochemistry. Third ed. Taylor & Francis Group: New York.
- Lehninger, A. L., Nelson, D. L., & Cox, M. M. (2000). Lehninger principles of biochemistry. New York: Worth Publishers.
- Biochemistry, Glycogenolysis – https://www.ncbi.nlm.nih.gov/books/NBK554417/
- Glycogenolysis – https://byjus.com/neet/glycogenolysis/
- Glycogenolysis – https://www.britannica.com/science/glycogenolysis
- Glycogenolysis – https://www.sciencedirect.com/topics/medicine-and-dentistry/glycogenolysis
- Glycogenolysis – https://biologydictionary.net/glycogenolysis/
- 5.1: Gluconeogenesis and glycogenolysis – https://med.libretexts.org/Bookshelves/Basic_Science/Cell_Biology_Genetics_and_Biochemistry_for_Pre-Clinical_Students/05%3A_Fuel_for_Later/5.01%3A_Glucone
- ogenesis_and_glycogenolysis
- Glycogenolysis – https://www.biologyonline.com/dictionary/glycogenolysis
- Regulation of Glycogenesis and Glycogenolysis – Biochemistry – https://www.pharmacy180.com/article/regulation-of-glycogenesis-and-glycogenolysis-1886/
Well explained