The field of bioengineering integrates natural sciences and engineering sciences to utilize organisms, cells, and molecular analogs to improve products and services. Biological systems are integrated with chemical engineering principles to produce the desired product.
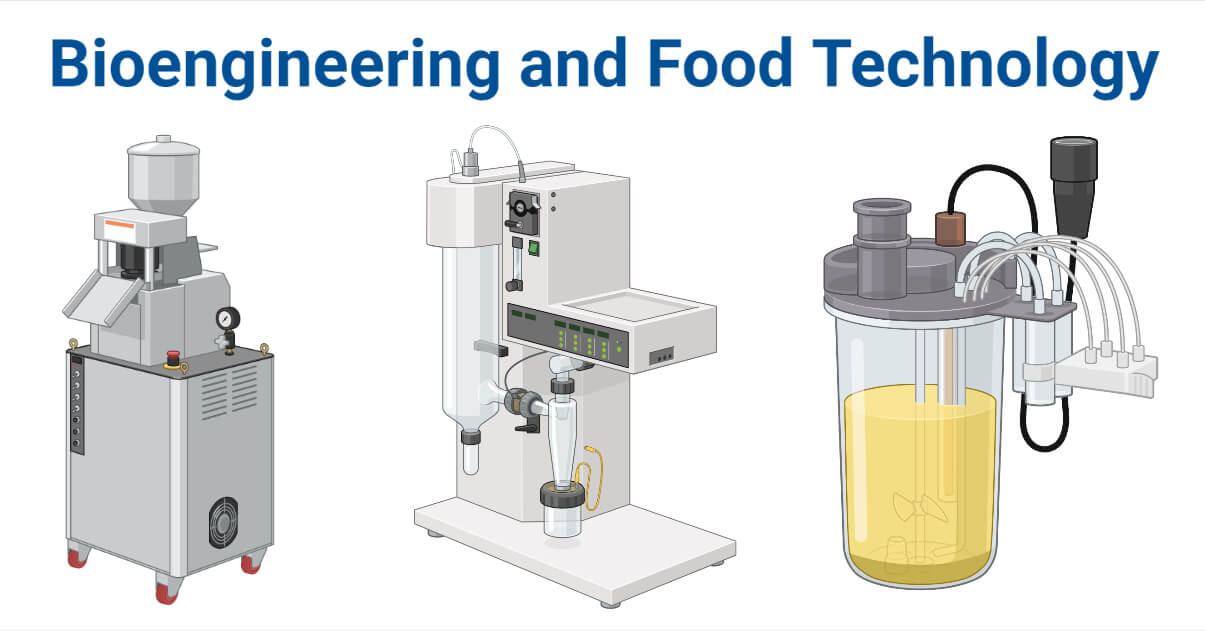
It also refers to applying technical knowledge and scientific principles to design and implement materials, structures, machines, devices, systems, and processes to achieve the desired objective.
In biochemical engineering, oxygenating aerobic fermentation processes sufficiently remains a challenge. Oxygen demands in high-density cultures far exceed the oxygen transfer capacity of conventional bioreactors.
Interesting Science Videos
Bioengineering and Bioreactor
- A bioreactor is a vessel designed specifically for growing microorganisms at high concentrations and providing the optimum conditions for their growth.
- A bioreactor is usually a cylindrical vessel with a hemispherical top and bottom, made of stainless steel or glass, ranging from a few liters to a few cubic meters.
- The sizes of the bioreactor can vary. The microbial cell (few mm3), shake flask (100-1000 ml), laboratory fermenter (1 – 50 L), pilot scale (0.3 – 10m3), to plant scale (2 – 500 m3 ) are all examples of bioreactors.
- Large-volume and low-value products like alcoholic beverages need simple fermenters and do not need aseptic conditions.
- High-value and low-volume products require a more elaborate system of operation and aseptic conditions.
- Bioengineering helps to build the optimal fermentation system for every microbe according to its process requirements.
- Stainless steel fermenters with capacities ranging from 30 to 50,000 liters are designed and built by Bioengineering for microbial cultivation (bacteria, archaea, yeast, and extremophiles).
- Reaction rates are usually expressed as the concentration of reactant consumed or the concentration of product formed per unit of time.
- The units are thus moles per liter per unit time, written as M/s, M/min, or M/h.
Calculating the Reaction Rate of the fermentation of sucrose to ethanol and carbon dioxide:
Rate=Δ [B]/Δt = −Δ [A]/Δt
C12H22O11 (aq) sucrose + H2O (l) → 4C2H5OH (aq) + 4CO2 (g)
Based on the coefficients in the balanced chemical equation, the reaction rate at which ethanol is formed is four times faster than the reaction rate at which sucrose is consumed:
Δ [C2H5OH]/Δt = −4Δ [sucrose]/Δt
- The concentration of the reactant, in this case, sucrose, decreases with time, so the value of Δ [sucrose] is negative.
- Consequently, a minus sign is inserted in front of Δ [sucrose] in the Equation, so the rate of change of the sucrose concentration is expressed as a positive value.
- Conversely, the ethanol concentration increases with time, so its rate of change is automatically expressed as a positive value.
- The reaction rate is often expressed in terms of the reactant or product with the smallest coefficient in the balanced chemical equation.
The smallest coefficient in the sucrose fermentation reaction corresponds to sucrose, so the reaction rate is generally defined as follows:
Rate=−Δ [sucrose]/Δt =1/4(Δ [C2H5OH]/Δt)
Equilibrium
Chemical reactions that can go both ways are indicated by the double arrows used in formulas.
When the rate of the reaction is the same in both directions, the reaction is said to be at equilibrium.
- Effects on Temperature
- When the temperature is increased, molecules have higher kinetic energy, which increases collisions between them and the amount of activation energy, so the reaction proceeds more quickly.
- Higher temperatures speed up fermentation because chemical reactions are involved.
- Effects on equilibrium.
- Enzymes are proteins that act as catalysts, examples in wine include: the enzymes that cause browning in juice are polyphenol oxidases such as lactase or tyrosinase.
- Enzymes are added to juice by winemakers to break down pectin in the juice to facilitate settling and clarification.
- The Enzyme lactase breaks down the milk sugar lactose, and people who lack it have lactose intolerance.
- Tartaric acid disassociates (loses its protons) in water Influences on Fermentation Temperature the lower the temperature, the slower the rate of fermentation, (In whites the ideal rate is about 1°B per day, reds 3-4 °B at peak of fermentation)
- If it gets too cold the rate slows to almost nothing.
- If it gets too hot (95 to 100 °F) the yeast can be killed and the fermentation will stick (stop).
- Effect of oxygen
- Microorganisms demand oxygen from the fermentor, while the fermentor provides it. During fermentation, the dissolved oxygen concentration should not fall below the critical dissolved oxygen concentration.
- Therefore, the oxygen-transfer rate of the fermentor matches the oxygen uptake rate of the culture while maintaining dissolved cellular concentration.
- Fermentors have a practical maximum KL, which is determined by the operating conditions of the fermentation; thus, to balance the supply and the demand. This may be achieved by:
- Controlling biomass concentration.
- Controlling the specific oxygen uptake rate.
- A combination of (1) and (2).
- Growth extents and mass of cells are determined by glucose levels; growth rates are influenced by oxygen dissolved and DO levels. The transfer of oxygen from gas bubbles to cells is limited by oxygen transfer through liquid film surrounding the gas bubbles;
NO2= kLa (C∗ −CL)
Where NO2 is the oxygen transfer rate (OTR) with the units of mgO2·l−1h−1 and;
• kL: O2 transfer coefficient (cm·h−1)
• a: gas-liquid interface area (cm2·cm−3)
• kLa: volumetric O2 transfer coefficient (h−1)
• C∗: saturated dissolved oxygen (mg·l−1)
• CL: actual dissolved oxygen (mg·l−1)
- Influences on Fermentation.
- Fermentation also produces heat as a byproduct; if no heat is lost the temp rises 2.3 °F for each drop of 1°B.
- Therefore, cooling jackets are used on tanks.
- The growth rate of yeast will double for each rise of 10°F.
- Yeast and bacteria (including spoilage microbes) grow best at about 5.5 pH.
- To prevent spoilage, keep must at 2.9 to 3.6 pH. Yeast grows slower at lower pHs.
- SO2 slows the growth of all microbes and kills some.
- To discourage non-wine yeasts and lactobacillus, add 30 to 50 PPM to the must
- Heat Transfer in Fermentation
- Two types of typical heat transfer applications in bioreactor operation.
- In situ batch sterilization of liquid medium. In this process, the fermenter vessel containing the medium is heated using steam and held at the sterilization temperature for a while; cooling water is then used to bring the temperature back to normal operating conditions
- Temperature control during reactor operation. The metabolic activity of cells generates heat.
- Some microorganisms need extreme temperature conditions (e.g. psychrophilic, thermophilic microorganisms) Heat transfer configurations for bioreactors: jacketed vessel, external coil, internal helical coil, internal baffle-type coil, and external heat exchanger.
- External jacket and coil give low heat transfer area. Thus, they are rarely used on an industrial scale.
- Internal coils are frequently used in production vessels; the coils can be operated with liquid velocity and give a relatively large heat transfer area.
- But the coil interferes with the mixing in the vessel and makes cleaning the reactor difficult.
- Another problem is the film growth of cells on the heat transfer surface.
- The external heat exchanger unit is independent of the reactor, easy to scale up, and provides the best heat transfer capability.
- However, conditions of sterility must be met, the cells must be able to withstand the shear forces imposed during pumping, and in aerobic fermentation, and the residence time in the heat exchanger must be small enough to ensure the medium does not become depleted of oxygen.
- Double-pipe heat exchanger.
- Shell and tube heat exchanger
- Plate heat exchanger
- Spiral heat exchanger
In bioprocess, the temperature difference is relatively small. Thus, a plate heat exchanger is rarely being used.
- Mass transfer in Fermentation
- Mass transfer occurs in mixtures containing local concentration variation.
- For example, when the dye is dropped into a cup of water, mass-transfer processes are responsible for the movement of dye molecules through the water until equilibrium is established and the concentration is uniform.
- Mass is transferred from one place to another under a concentration difference or concentration gradient in the system.
- Gas-liquid mass transfer is extremely important in bioprocessing because many processes are aerobic. Oxygen must first be transferred from gas bulk through a series of steps onto the surfaces of cells before it can be utilized.
- The solubility of oxygen within broth is very poor. Therefore, the enhancement of gas-liquid mass transfer during aerobic cultures and fermentations is always put into priority.
Applications of Bioengineering
Different bacteria have different process requirements – Bioengineering builds the optimal fermentation system for every microorganism.
- Bioengineering designs and builds customized stainless steel fermenters for microbial cultivation (bacteria, archaea, yeast, and extremophiles) with capacities from 30 to 50,000 liters.
- Oxygen supply, stirrer dimensioning, heating, and cooling circuit. These are critical factors for challenging microbial cultures. Often, a high material transition coefficient (kLa value) must be achieved with high power input. Bioengineering can support you with expert knowledge and CFD simulations.
- With over 40 years of experience, Bioengineering develops aeration strategies, ensuring optimal oxygen supply for your microorganism and process.
- Anaerobic or with high oxygen demand, high foam development, bio-safety requirements, high metabolic heat, continuous fermentation, GMP production, high cell density – you name the issue; Bioengineering will come up with the solution.
- Dosage vessels for corrective agents such as acids, bases, and anti-foams are standard system features and are fully integrated into the fermentation system.
- Short heating and cooling periods are a priority for us to avoid negative effects on culture media and process duration.
References
- Bauer, W., & Durbeck, E. (1990). Bioengineering in food technology. Food Biotechnology, 4(1), 123–136. https://doi.org/10.1080/08905439009549728
- Bérangère, F., & Laurent, P. (2012). Methodology for Bioprocess Analysis : Mass Balances , Yields and Stoichiometries. i.
- Gaden, E. L. (1959). Microbiological Process Discussion Bioengineering and Fermentation. Bioengineering and Fermentation, 8, 123–131.
- González-Figueredo, C., Flores-Estrella, R. A., & Rojas-Rejón, O. A. (2018). Fermentation: Metabolism, Kinetic Models, and Bioprocessing. Current Topics in Biochemical Engineering. https://doi.org/10.5772/INTECHOPEN.82195
- Jarvis, W. B. (2008). Science & Technology Libraries A Brief Fermentation Biotechnology Guide to Biochemical Engineering, Industrial Microbiology and Fermentation Literature. https://doi.org/10.1300/J122v05n01_11
- Knoll, A., Maier, B., Tscherrig, · Hermann, & Büchs, J. (2005). The Oxygen Mass Transfer, Carbon Dioxide Inhibition, Heat Removal, and the Energy and Cost Efficiencies of High-Pressure Fermentation. https://doi.org/10.1007/b98918
- Lavric, V. (2016). Bioreactors in Biomedical Engineering. An Introduction Bioreactors in Biomedical Engineering. An Introduction. January 2017.
- Uzir, MH., Don, MM.(2007). Biochemical engineering. Retrieved November 15, 2021, from http://chemical.eng.usm.my/notes/HEKARL/notes/ekc471_notes.pdf
- Prokop, A. (2008). Bioengineering Problems Connected with the Use of Conventional and Unconventional Raw Materials in Fermentation. 69, 1–12. papers2://publication/UUID/7C321D0B-1FA1-43E2-B065-044C00FB115C
- Renewable, N. (2012). “BEST”: Biochemical Engineering Simulation Technology. D, 1–23. https://www.nrel.gov/docs/legosti/old/20548.pdf%0Apapers3://publication/uuid/E05ABAAC-35D3-422C-B951-85254975E161
- Sivasubramaniam, D., & Franks, A. E. (2016). Bioengineering microbial communities: Their potential to help, hinder and disgust. Bioengineered, 7(3), 137. https://doi.org/10.1080/21655979.2016.1187346
- Yoshida, T. (2017). Biocatalysts and Enzyme Micro- and Nanosystems for Culture of Living Cells Manufacturing of Further Volumes of the Series.