The cellular membrane of biological organisms is semi-permeable in nature, meaning they allow only a fraction of ions, molecules, and water across a membrane. The permeability of these molecules varies based on the structural pattern of lipid bilayer among different types of cells and organisms. The lipid bilayer, in accordance with macromolecules such as proteins, carbohydrates, and lipids, facilitates the transport of molecules. The ability of any membrane to control the transportation of molecules across a membrane is considered as one of the essential life processes in an organism.
The membranes apply two modes of transport for molecular movement, this includes:
Passive Transport – This transport mechanism involves the movement of substances across the membrane down the concentration gradient from high to low without employing any energy expenditure.
Examples include:
- The diffusion of neurotransmitters like acetylcholine across synaptical junctions in neurons.
- Diffusion of gases in the lungs across alveolar membranes.
- Movement of water molecules by osmosis.
Active Transport – A transport mechanism that involves the movement of molecules across the membrane against the concentration gradient, meaning the transport of substances from low to high concentration. This movement is achieved by the energy expenditure from ATP (adenosine triphosphate).
Examples include:
- Movement of Ca2+ ions out of the cardiac muscle cells.
- Transportation of glucose molecules across membranes.
- Movement of amino acids in the human gut across the intestinal lining.
Read Also- Active vs passive transport- Definition, 18 Differences, Examples
Any mutational changes in the transportation system or the membrane transporters involved may lead to severe health conditions like depression, cystic fibrosis, drug abuse, migraine, and epilepsy.
Interesting Science Videos
Lipid Bilayers and Membrane Proteins
- Most biological membranes are composed of a phospholipid bilayer, with the hydrophilic side exposed outwards and the hydrophobic side facing inwards, and transmembrane proteins spanning the bilayer.
- The integrated proteins present in the membrane form the transport channel, pumps, and carriers that are required for the translocation of ions and macromolecules across biological membranes.
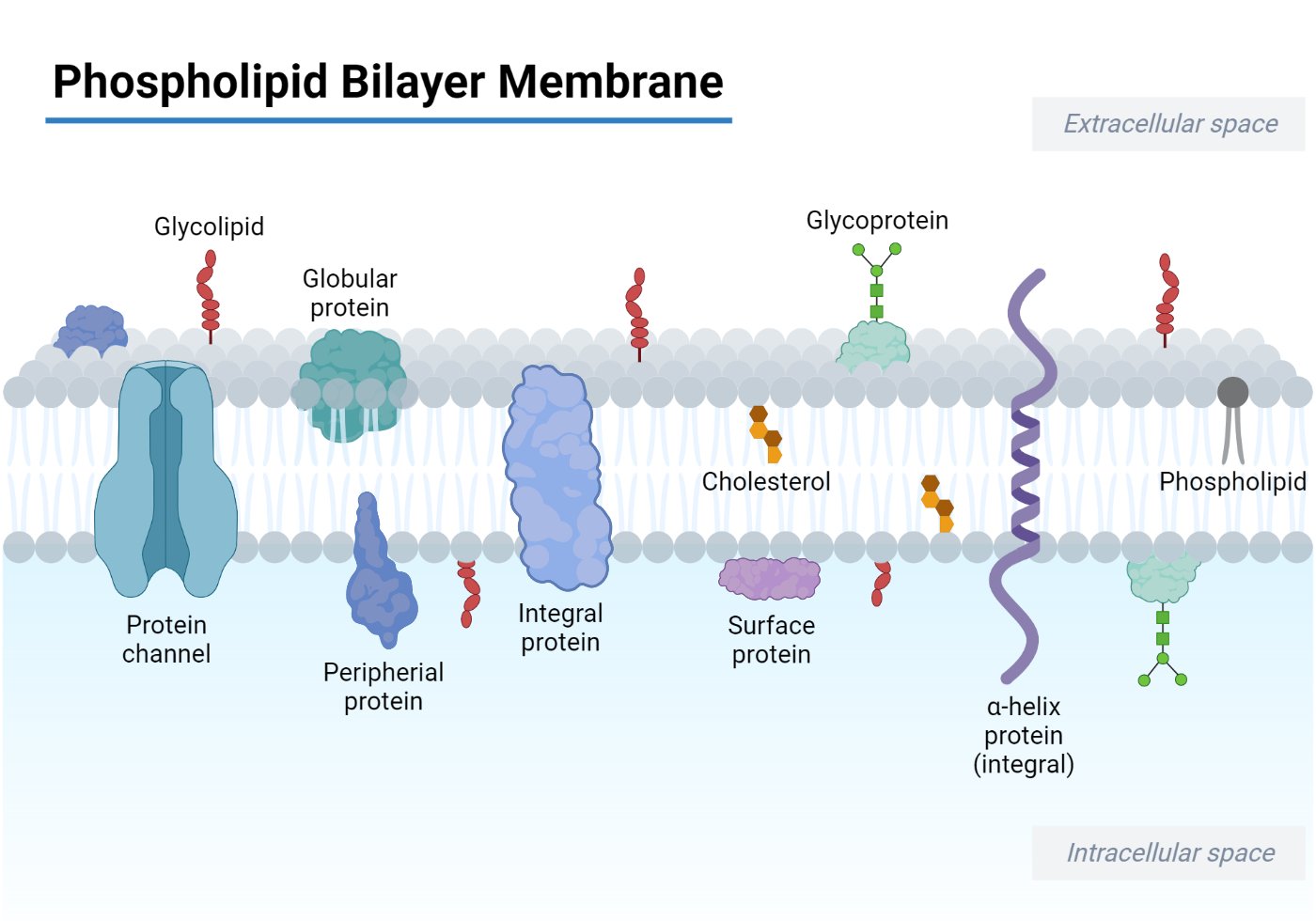
- Channel proteins form pores in the membrane to allow free passage of inorganic ions like Na+, Cl-, K+, H+, etc, and molecules of appropriate size.
- The channel proteins are strictly regulated by extracellular signals to control the movement of ions and solutes across the membrane.
- Carrier proteins act like an enzyme that selectively binds to and transports specific small molecules such as glucose to facilitate the translocation across the membrane.
- The composition of the phospholipid-to-protein ratio varies depending on the type of cell it is present in. For example, the mitochondrial inner membrane has 75% protein suggesting the presence of protein complexes involved in oxidative phosphorylation and the electron transport system.
What is Active Transport?
Active transport is the energy-driven transportation of ions, small molecules, and solutes across the biological membrane against an electrochemical gradient (for ions) or concentration gradient i.e., from lower to higher concentration.
Since this mode of cellular transportation requires energy, a significant amount of cellular energy is spent on regulating the homeostasis of molecules and ions in a cell. Active transport is further divided into two types – Primary and Secondary active transport.
Active Transport Types
Classification of Active Transport is based on how energy is employed to bring about the movement of substances. Primary active transport is seen to use ATP as an energy source to translocate solutes against a concentration gradient, whereas in Secondary active transport, an electrochemical gradient is applied.
Primary Active Transport
This category of active transport directly employs the use of metabolic energy to translocate substances across the membrane, hence also called as ‘Direct Active Transport.’ The transportation of molecules uses energy released from ATP, where Adenosine triphosphate (ATP) is broken down to ADP (adenosine diphosphate). This transport mode is used to move metal ions like K+, Na+, Cl-, H+, Mg2+, and Ca2+. The enzymes utilized for the primary active transport of ions are ATPases that facilitate the movement of charged ions across ion channels or pumps.
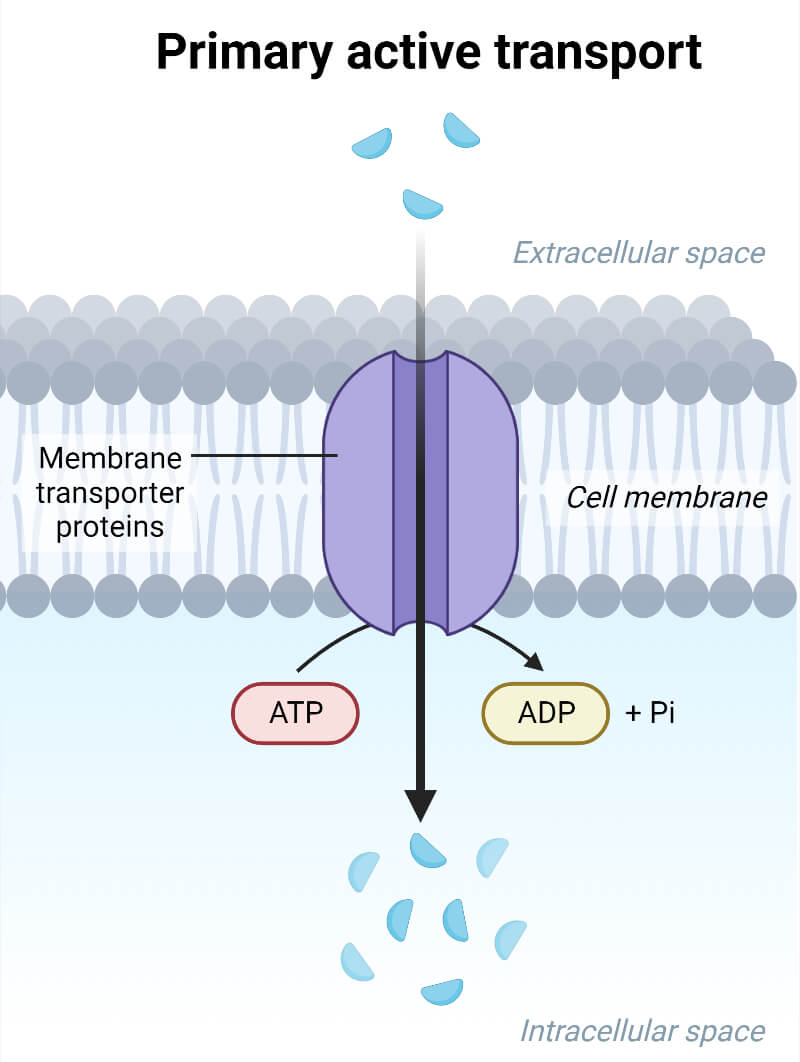
Types of Primary Active Transport
P-Type ATPases
They are also referred to as E1-E2 (enzyme1-enzyme2) ATPases due to their ability to interchange between two conformations.
- In humans, the pumps with P-type ATPases show diverse roles in nerve impulses, absorption of nutrients and solutes in the intestine and kidney, and in relaxation of muscles.
- Eukaryotes and bacteria have ion channels with P-type ATPases.
- The ‘P-type’ refers to the autophosphorylation among components present in the pumps, such as ATP and aspartate, present in the enzyme structural composition.
- The enzyme structure typically contains four significant functional domains to carry out the transportation process, which include
- P-domain (Phosphorylation) containing amino acids like aspartate.
- N-domain – Used for nucleotide binding.
- A-domain – Actuator domain which contains a phosphatase domain for dephosphorylation of the phosphorylated element.
- R-domain – Regulatory domain.
Examples of P-type ATPases involved in ions pumps include Na+-K+ (sodium-potassium ) ion channel, Calcium ATPase (Ca2+ pump), Hydrogen ATPase (H+ pump), and H+/K+ ATPase in proton-potassium pump, in fungi, bacteria, humans, and plants.
Mechanism of Sodium-Potassium (Na+/K+ ATPase) Pump
This pump employs a direct utilization of ATP to bring about the conformational changes to the protein and allow passage of three Na+ ions out of cells while bringing two K+ ions into the cell.
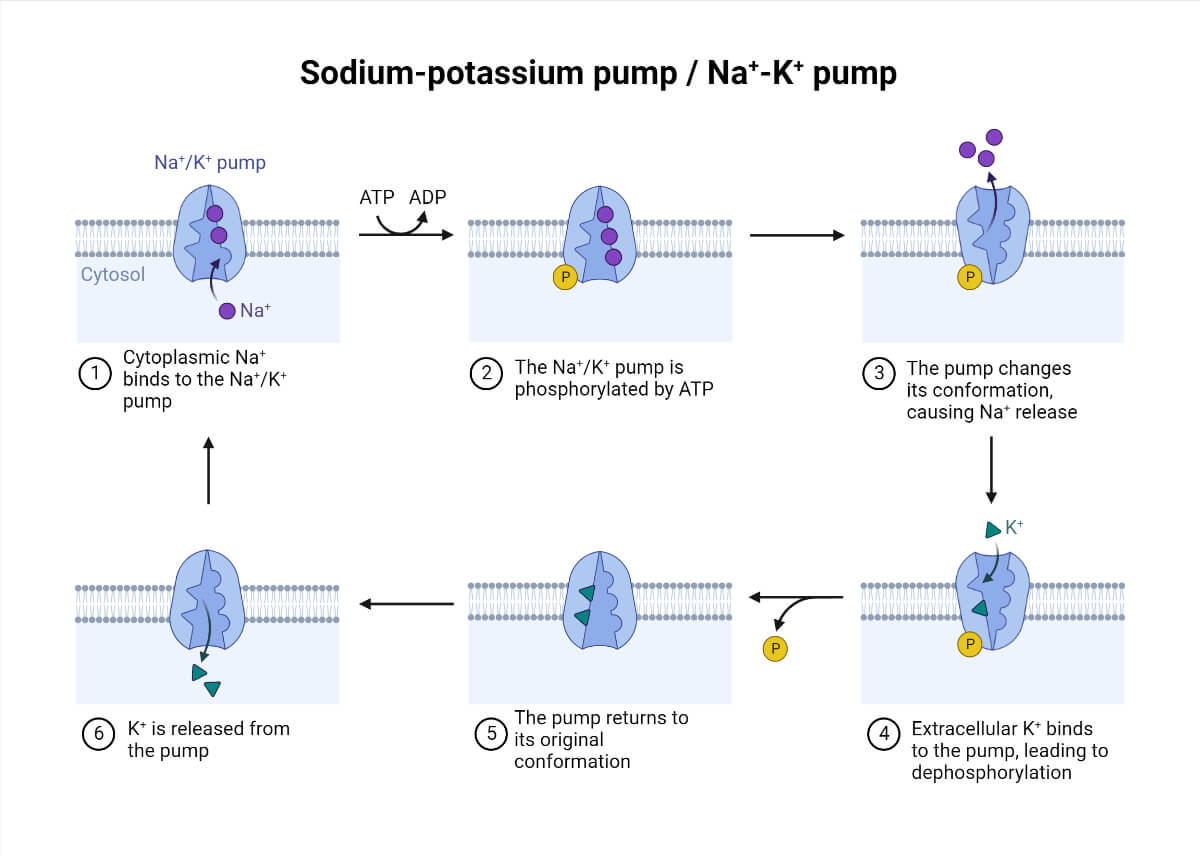
- The transmembrane carrier protein is opened towards the cell interior with a high affinity for sodium ions.
- The binding of sodium ions to the carrier protein induces phosphorylation of the transmembrane protein by ATP hydrolysis.
- The phosphorylation of protein modifies the structural conformation making it open to the cell exterior, leading to a lower affinity for sodium ions, hence releasing the three bound sodium to the extracellular space.
- The outward-facing carrier protein conformation shows a higher affinity for potassium ions.
- As the potassium ions bind to the carrier protein, it releases the phosphate group attached to the protein.
- The detachment of the phosphate group from the protein causes it to change to its original conformation facing to the cell interior.
- The change in conformation loses the affinity for potassium ions, hence releasing it into the cell, and again sodium ions can bind to the protein, and the cycle repeats continuously.
- A cardiac glycoside called ‘Ouabain’ blocks the influx of potassium ions into the cell by binding to the protein surface and inhibiting the dephosphorylation of carrier protein.
F-ATPase
They are mainly found in the mitochondrial inner membranes and chloroplast’s thylakoid membrane.
- They are sometimes also referred to as ATP phosphohydrolase that transports H+ ions, or as ATP synthase.
- The enzyme uses rotating machinery to pump Na+ or H+ ions which are powered by energy derived from ATP hydrolysis.
- F-type ATPase contains two main domains – F1 and F0.
- F1 Domain – It is a Hydrophilic catalytic globular domain made of an asymmetric hexameric ring and a central stalk at the center of the ring. This domain contains catalytic sites for ATP hydrolysis and synthesis.
- F0 Domain – Responsible for the translocation of ions across a membrane. It is hydrophobic in nature and is embedded in the membrane.
- The energy obtained from the movement of protons across the membrane is used to drive ATP synthesis, which helps in releasing newly formed ATP from F-ATPase’s active site.
- ATP hydrolysis is done at conditions of a lower driving force. Hence ATPase functions as ATP synthase that generates a transmembrane electrochemical gradient.
V-ATPase
The V denotes vacuolar ATPases, which are proton-translocating pumps present in the tonoplast of cells. They are responsible for making the organelle more acidic than the cytoplasm around them.
- This enzyme employs the energy obtained from ATP hydrolysis to drive the transport of protons across biological membranes.
- It is a multimeric protein that uses rotatory machinery to allow the passage of ions and has two distinct domains – V1 and V0.
- V1 Domain – Contains catalytic site for ATP hydrolysis and comprises eight subunits.
- V0 Domain – This domain is responsible for the movement of protons across a membrane.
- They are considered an important molecular switch that toggles between anabolic and catabolic metabolism, and any disruption to their structure can lead to diseases like cancer.
ATP Binding Cassette (ABC) Transporters
They are significantly found in chloroplasts, mitochondria, and plasma membranes and are seen to play a vital role in detoxification, phytohormone transport, and in pathogen response. They utilize the energy released from the hydrolysis of ATP to allow the passage of solutes across a membrane.
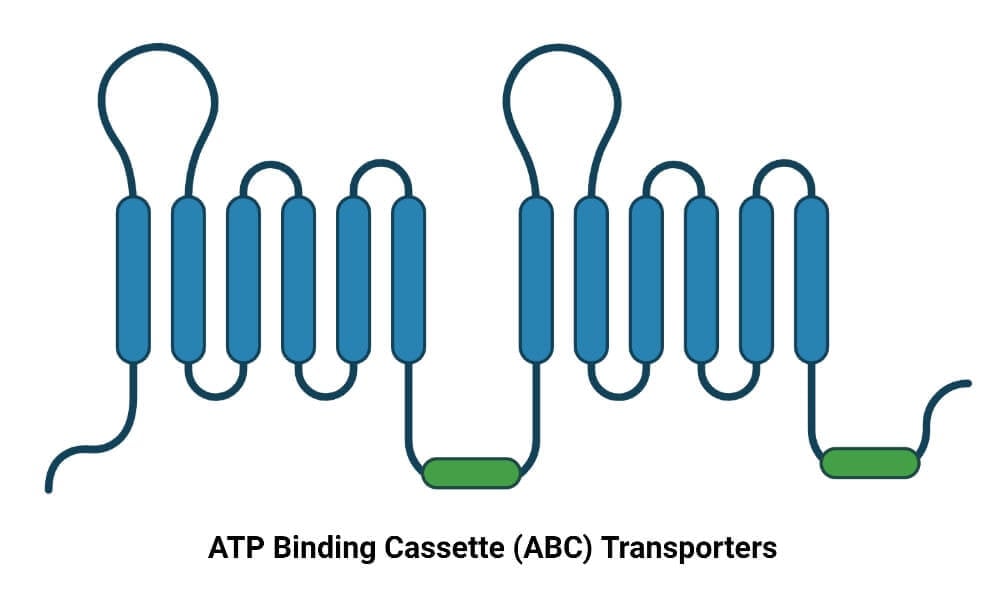
- They have four significant domains, two of which are transmembrane integral domains spanning the membrane six times, and the other two domains are responsible for ATP hydrolysis.
- They are actively seen to export antimicrobial metabolites and volatile compounds such as benzene, 1,3-butadiene, etc.
- Approximately 48 genes are identified in humans, and most of these genes are part of diseases like Dubin-Johnson Syndrome, Cystic Fibrosis, Ataxia, Anemia, adrenoleukodystrophy, and many more.
- They participate in processes like drug/antibiotic resistance, signal transduction, antigen presentation, bacterial pathogenesis, etc.
Secondary Active Transport
Also called as Coupled Transport or Cotransport, as there is a movement of ions along with large molecules like glucose. First, the ions are pumped down the electrochemical gradient but against a concentration gradient, as a result of which energy is released. Then the solute is translocated across a membrane down the concentration gradient.
- The functions performed by this mode of transport include the generation of an electrochemical gradient due to the movement of ions at the expense of energy across a membrane.
- The ion gradient difference leads to a movement of solute in either the opposite direction, referred to as Antiport, or in the same direction, referred to as Symport.
- In yeast and bacteria, hydrogen (H+) ions are the commonly cotransported ion, whereas, in humans, sodium (Na+) ions are widely used for coupled transportation.
Secondary Active Transport Types
There are basically two modes of secondary active transport based on the direction of solute along with ions across a membrane: Antiport (opposite direction) and Symport (same direction). The level of effective movement of substances can be measured by the concentration capacity of the transporters to translocate ions/solutes in the process per cycle. The higher the ion/substrate coupling ratio, the higher the concentration capacity of the transporter. For instance, the coupling of sodium (Na+) ions to glucose results in the movement of two sodium ions to one glucose molecule per transport cycle with a ratio of 2:1.
Antiport
Also referred to as Counter transport or Exchanger as solutes and ions are allowed to move in the opposite direction. Examples include the Na+/Ca2+ transporter via calcium ATPase, Cl-/HCO3- transporter, and Na+/H+ transporter.
- Na+/Ca2+ Antiporter – This antiporter is necessary to regulate low calcium concentration in the cardiac muscle cells. This exchanger allows three Na+ ions down the electrochemical gradient and one Ca2+ ions against the electrochemical gradient from low to high solute concentration with a stoichiometric coupling ratio of antiporter 3:1.
- Cl-/HCO3- Antiporter – Results in electroneutral exchange as one Cl- ion down its electrochemical gradient per bicarbonate (HCO3-) ion against the electrochemical gradient with a stoichiometric coupling ratio of antiporter 1:1.
- Na+/H+ Antiporter – Electroneutral mechanism and very useful in maintaining the cytoplasmic pH as one Na+ ion is transported down its electrochemical gradient per H+ ion against its gradient with a stoichiometric coupling ratio of antiporter 1:1.
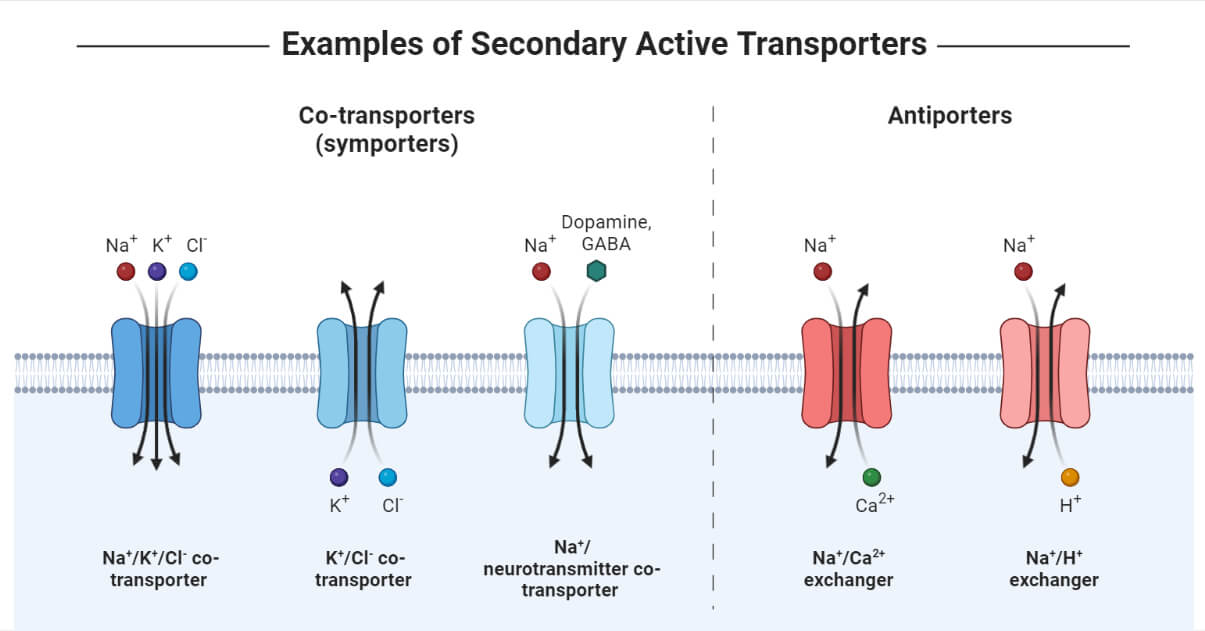
Symporter
The solutes and ions are transported in the same direction, with one substance being translocated down its concentration gradient while the other substance is moved against its gradient from low to high solute concentration. Examples include glucose symporter (SGLT1), GABA symporter (GAT), oligopeptide symporter (PepT), and more.
- Glucose symporter (SGLT1) – It can be found in the cells of the intestinal epithelium, nephrons of the kidney, brain, and in heart. Two molecules of Na+ ions are imported down its concentration gradient into the cell per glucose (or galactose) molecule against the gradient.
- GAT Symporter – Responsible for regulating the concentration of GABA (gamma-aminobutyric acid), an inhibitory neurotransmitter in the synaptic cleft of the nervous system. The symporter is coupled with Na+ or Cl- ions for solute movement across a membrane.
- Oligopeptide symporter (PepT) – Serves as a crucial entry route for drugs like beta-lactam antibiotics and helps in the reabsorption of nitrogen in the intestine and kidney. In this symporter, one H+ ion is transported from high to low concentration per dipeptide or tripeptide molecule against its concentration gradient.
Conclusion
Active transport is a crucial transport mechanism opted by cells to maintain ionic homeostasis in the cytoplasm and regulate the uptake/removal of drugs, nutrients, and waste products. This is an energy-driven process of translocating substances across a membrane down or against their electrochemical and concentration gradient. Active transport is categorized into two modes which include primary active transport (directly employs ATP’s energy to drive ions across the membrane) and secondary active transport (using energy from electrochemical gradient to couple movement of solutes and ions). It is a highly specific and regulated process with multiple proteins and transporter working together to allow efficient passage of molecules. Any anomalies in the structure of a transporter affect its efficacy in delivering molecules across the membrane, which leads to diseases like cancer, cystic fibrosis, renal tubular acidosis, Barter syndrome, and many more.
References
- Physiology, Active Transport – https://www.ncbi.nlm.nih.gov/books/NBK547718/
- Active transport – https://en.wikipedia.org/wiki/Active_transport
- Active Transport: Definition, Types, and Examples – https://conductscience.com/active-transport-definition-types-and-examples/
- Active transport – https://www.biologyonline.com/dictionary/active-transport
- Active Transport – https://www.sciencedirect.com/topics/immunology-and-microbiology/active-transport
- Cell Membranes – https://www.ncbi.nlm.nih.gov/books/NBK9928/
- The Lipid Bilayer – https://www.ncbi.nlm.nih.gov/books/NBK26871/
- P-Type ATPase – https://www.sciencedirect.com/topics/biochemistry-genetics-and-molecular-biology/p-type-atpase
- https://www.frontiersin.org/articles/10.3389/fphys.2019.00358/full
Thanks for this awesome answer